

HS Chiong1, YK Yong2, MS Mohd Hijaz1, MR Sulaiman1 , KH Yuen3 and MN Hakim1,4,5*
1Department of Biomedical Sciences, Faculty of Medicine and Health Sciences, Universiti Putra Malaysia, UPM Serdang, Selangor, Malaysia. 2Department of Human Anatomy, Faculty of Medicine and Health Sciences, Universiti Putra Malaysia, UPM Serdang, Selangor, Malaysia. 3Discipline of Pharmaceutical Technology, School of Pharmaceutical Sciences, Universiti Sains Malaysia, Minden, Penang, Malaysia. 4Halal Product Research Institute, Universiti Putra Malaysia, UPM Serdang, Selangor, Malaysia. 5Institute of Bioscience, Universiti Putra Malaysia, UPM Serdang, Selangor, Malaysia..
DOI : https://dx.doi.org/10.13005/bpj/2905
Abstract
Piroxicam, a nonsteroidal anti-inflammatory drug, has been shown with low oral bioavailability and delayed onset of its therapeutic effects. In this work, a promising nano/liposomal drug delivery system was exploited to improve the in vivo therapeutic efficacies of piroxicam. The current liposome-encapsulated piroxicam formulation effectively boosted and prolonged peripherally mediated anti-nociceptive activities in tests for abdominal writhing induced by acetic acid (inhibition of pain 70.19% was in mice treated with 30 mg/kg liposome-encapsulated piroxicam), paw licking induced by formalin (81.36% inhibition when compared to free unencapsulated piroxicam), and hyperalgesia induced by carrageenan (55.8% inhibition when compared to free unencapsulated piroxicam). Even lower dose of liposomes-encapsulated piroxicam was also significantly inhibit Brewer’s yeast-induced hyperthermia. Carrageenan-induced paw-edema test and cotton pellet-induced granuloma test revealed that liposomes-encapsulated piroxicam had significantly more potent acute and chronic anti-inflammatory effects than piroxicam, even if lower drug dosages were used to treat animals. A better modulation in the generation of inflammatory mediators (nitric oxide, tumour necrosis factor-α, interleukin-1β, and interleukin-10) at 18.02% (TNFa), 23.97% (IL-1β) and 10.27% (IL-10) inhibition when compared to 30mg/kg free piroxicam group respectively. was ascribed to the higher in vivo therapeutic actions. Present nano-encapsulated piroxicam also significantly enhanced the inhibition of cyclooxgenase-2 (total percentage inhibition was increased by 18.25% and 19.22% at drug dosage of 3 and 30 mg/kg, respectively), but not cyclooxgenase-1 enzyme. In conclusion, present study showed that liposomal drug formulation was able to improve the in vivo therapeutic effects of orally administered piroxicam.
Keywords
Anti-inflammation; Anti-nociceptive; Anti-pyretic; Liposome; Piroxicam
Download this article as:
Copy the following to cite this article: Chiong H. S, Yong Y. K, Hijaz M. S. M, Sulaiman M. R, Yuen K. H, Hakim M. N. Improved Anti-nociceptive, Anti-pyretic and Anti-inflammatory Effects of Orally Administered Liposome-encapsulated Piroxicam. Biomed Pharmacol J 2024;17(2). |
Copy the following to cite this URL: Chiong H. S, Yong Y. K, Hijaz M. S. M, Sulaiman M. R, Yuen K. H, Hakim M. N. Improved Anti-nociceptive, Anti-pyretic and Anti-inflammatory Effects of Orally Administered Liposome-encapsulated Piroxicam. Biomed Pharmacol J 2024;17(2). Available from: https://bit.ly/4cdKfyq |
Introduction
Piroxicam is a popular non-steroidal anti-inflammatory drug (NSAID) that exhibits prominent anti-nociceptive, anti-pyretic and anti-inflammatory activities1,2. This class II drug of Biopharmaceutical Classification System, characterized by low solubility and high permeability, is widely used for acute or long-term treatment of various musculoskeletal and joint disorders3-5. Hence, rapid and sustainable drug effects to relief the signs and symptoms in these inflammatory diseases are highly desirable6, 7.
The dissolution of piroxicam in vivo, however, is known to be an absorption rate-limiting step which critically affects its therapeutic activities3,8. Previous pharmacokinetic study has revealed that this poorly water-soluble drug, when orally administered, requires more than two hours to attain a peak plasma concentration. The slow absorption rate renders a low bioavailability of drug and delayed the onset of its therapeutic effects9-11. Attempts to increase treatment efficiency by escalating the administered dose, however, increase risk of serious adverse drug reactions12. Due to these drawbacks, it is therefore important to develop a new oral piroxicam formulation with enhanced onset of action and stronger therapeutic activities.
Liposomal drug delivery system appears to be an ideal approach to provide better therapeutic efficacy over an existing drug formulation, mainly through alteration in dissolution and biodistribution of entrapped drug13-16. Liposomes-encapsulated piroxicam formulations have been previously reported to increase topical chronic anti-inflammatory activity in experimental animal by as much as 26.3%1, 17. We also successfully demonstrated the effectiveness of liposomal drug-encapsulation strategy to improve anti-inflammatory effects of piroxicam in vitro18. The current study was therefore intrigued to evaluate the potential of present liposomal drug formulations in enhancing effectiveness of piroxicam in vivo. The pharmacological properties such as anti-nociceptive, anti-pyretic and anti-inflammatory effects as well as the underlying mechanisms exhibited by piroxicam and liposome-encapsulated piroxicam administered orally were investigated using various experimental animal models.
Materials and Methods
Materials
Pro-lipoTM Duo was from Lucas Meyer, France. Piroxicam, dimethylsulfoxide, carrageenan, Brewer’s yeast, tribromoethanol, ter-amylalcohol, sodium chloride, acetylsalicylic acid and lipopolysaccharide from Escherichia coli were purchased from Sigma, US. Acetic acid, ethanol and formalin were originated from BDH, UK. Diethyl ether was obtained from R&M Marketing, UK.
Preparation of liposomal samples
Liposomal samples were prepared at room temperature in accordance to previously described procedures19. Briefly, stock piroxicam solution (60 mg/mL dimethylsulfoxide) was added into Pro-lipoTM Duo with moderate stirring (125±25 rpm) for 60 minutes. Concentrated piroxicam-loaded liposomal suspension was formed by drop-wise addition of distilled water (dH2O). This liposomal suspension was continuously stirred for 10 hours before been further diluted with dH2O. Mixture was then stirred for 30 minutes. The ratio of stock piroxicam solution: Pro-lipoTM: dH2O (hydration): dH2O (dilution) was 1:5:9:25 w/w/w/w. Blank liposomes were prepared according to same procedure except that dimethylsulfoxide was used instead of stock piroxicam solution. Final concentration of dimethylsulfoxide in all prepared samples was 2.5%. The resulting mean liposomes diameter was around 370 nm with polydispersity index ranging from 0.4 to 0.5.
Experimental animals
There were 288 male Sprague Dawley rats weighing between 150 and 300 g and 48 male BALB/c albino strain mice weighing between 22 and 42 g. The mice were randomly assigned to normal cages with a light/dark cycle of 12 hours, a temperature of 25±2°C, and a humidity level of 70–80%. Every day, the animals were given access to tap water and a pelleted food. Before beginning any experimental modification, animals were given a minimum of seven days to acclimate. The Faculty of Medicine and Health Sciences, Universiti Putra Malaysia’s Animal Care and Use Committee gave approval for the experiments to be conducted (Ref. No. UPM/FPSK/PADS/UUH/F05).
Anti-nociceptive assays
Acetic acid-induced abdominal writhing test
The test was conducted in accordance with Sulaiman20 protocol description. Oral piroxicam or liposome-encapsulated piroxicam at 0, 0.3, 3, and 30 mg/kg was administered to mice (n = 6/group). Mice were given a 30-minute pre-treatment period, intraperitoneal injections of 0.6% acetic acid (10 mL/kg), and their enclosures made of clear Perspex. After a 5-minute delay, the presence of contraction of the abdominal muscles along with elongation of the body and extension of the limbs (writhing effect) was cumulatively counted at 5-minute intervals for a duration of 30 minutes. The following ratio could be used to indicate the percentage of protection in terms of writhing number (W):

Formalin-induced paw-licking test
Rats (n=6/group) were given an initial 20-minute accommodation period in an observation room, as per Mossadeq21 description, before receiving oral treatment with piroxicam or liposome-encapsulated piroxicam at 0, 0.3, 3, and 30 mg/kg. Thirty minutes before the intraplantar injection of a 2.5% formalin solution (50 µL), all treatments were completed. The length of time (T) that the animal licked or bit the injected paw—a sign of a pain reaction—was noted for both the early phase (0–5 minutes) and the late phase (15–30 minutes). The formula used to compute the percentage inhibition of licking was as follows:

Carrageenan-induced mechanical hyperalgesia test
According to Fujii22, mechanical hyperalgesia in unrestrained rats was quantified as the hind limb withdrawal threshold in response to a mechanical stimulus by the use of a modified Randall-Sellito test. A 0.1 mL intraplantar suspension of 1% carrageenan was used to elicit hyperalgesia in the hind paw 30 minutes after the rats (n = 6/group) received piroxicam or liposome-encapsulated piroxicam orally at 0, 0.3, 3, and 30 mg/kg. Using a dynamic plantar aesthesiometer (model 37450, Ugo Basile, Italy) fitted with a rounded-tip cone-shaped paw-presser that applied a linearly increasing upward force (20 g/s) to the plantar surface of the paw, the nociceptive response to pressure in the injected paw was assessed. The force (F) that made each animal remove its paw was the nociceptive threshold, measured in grams. Measurements were performed three times at several-second intervals, and mean value was taken as threshold. The reading was taken immediately after and at 1, 2, 3, 4 and 5 hour intervals following carrageenan injection. A cut-off of 300 g was used to prevent mechanically induced injury. All tested rats were habituated to the full procedure for three consecutive days prior to actual experimentation. The percentages of inhibition of forces recorded are calculated using formula stated below:

Carrageenan-induced thermal hyperalgesia test
Noxious heat stimulation of hind paw was assessed in unrestrained rats using the Hargreaves model of thermal hyperalgesia as described previously by Ortiz23. Rats (n=6/group) were first treated by oral administration of piroxicam or liposome-encapsulated piroxicam at 0, 0.3, 3, 30 mg/kg. After 30 minutes of pre-treatment, hyperalgesia was induced in the hind paw by intraplantar administration of 0.1 mL of 1% carrageenan suspension. Each animal was subjected to identical testing procedure using plantar test (model 37370, Ugo Basile, Italy). The source of the thermal nociceptive stimulus was an aluminum cylindrical vessel fitted with an infrared source. The plantar surface of the injected paw was manually targeted with a radiant infrared heat source (set to 50 i.r.) while the rat remained still. The light source automatically set off a timer, and the duration needed for a paw to exhibit an abrupt withdrawal was known as the paw withdrawal latency (L). To prevent tissue injury, a 20-second cutoff limit was placed on the stimulation duration. Paw withdrawal latencies were measured immediately following carrageenan injection, as well as at 1, 2, 3, 4, and 5 hours later. Three readings were taken at intervals of several seconds throughout each time point, and the mean value was used to determine the nociceptive threshold. Percentage of inhibition of thermal hyperalgesia was calculated using following formula:

Anti-pyretic assay
Brewer’s yeast-induced hyperthermia test
Rats were made hyperthermic via a technique outlined by Owoyele24. A digital thermometer with a lubricated probe was used to take the patient’s initial rectal temperature. Rats were given a subcutaneous injection (10 mL/kg) of a 20% brewer’s yeast slurry in the dorsum. For this investigation, only rats exhibiting a rectal temperature increase of at least 0.7 °C after 18 hours were employed. Oral piroxicam or liposome-encapsulated piroxicam at 0, 0.3, 3, and 30 mg/kg was administered to six randomly chosen rats per group. The rectal temperature (R) was taken up to five hours after the therapy was administered at one-hour intervals. The formula used to compute the percentage of inhibition of hyperthermia was as follows:

Anti-inflammatory assays
Carrageenan-induced paw-edema test
As stated by Mossadeq21, this test was conducted. Oral piroxicam or liposome-encapsulated piroxicam at 0, 0.3, 3, and 30 mg/kg was administered to rats (n = 6/group). Each rat’s hind paw received 0.1 mL of 1% carrageenan solution intraplantarly 30 minutes after treatment, resulting in acute inflammation (swollen paws). Paw volume was measured using plethysmometry (model 7140 plethysmometer, Ugo Basile, Italy) both immediately after (V0) and at 1, 2, 3, 4, and 5 hour intervals (VT) after carrageenan injection. The volume displaced by the paw between final volume (VT) and initial volume (V0) was used to measure the degree of inflammation. To ascertain the anti-inflammatory property, the percentage inhibition of edema was computed in relation to control.

Cotton pellet-induced granuloma test
The test was conducted according to Panthong25 instructions. First, six rats per group (10 mL/kg) were anesthetized with 2% tribromoethanol. Next, shaved dorsal portion of thoracic vertebrae region was surgically implanted with 30 ± 1 mg sterile cotton pellets per subcutaneous tissue. All rats received piroxicam or piroxicam liposome-encapsulated at 0, 0.3, 3, and 30 mg/kg orally throughout the seven-day study. Rats were killed on day eight by overdose on diethyl ether. After removing the cotton pellets, their wet weight was immediately determined. The cotton pellets were then weighed again after chilling and drying for an additional eighteen hours at 60 °C. The following formulas were used to determine the test compound’s transudative and granuloma weight as well as its percent transudative and granuloma inhibition:
Transudative weight (C) = Wet pellet weight – Dried pellet weight
Granuloma weight (G) = Dried pellet weight – Initial pellet weight
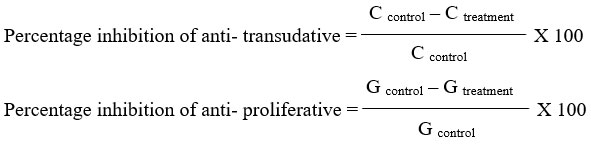
Assay of nitric oxide (NO), cytokines and cyclooxygenase (COX) activities
Blood samples from rats in the cotton pellet-induced granuloma test model were used in this study. During the last day of experiment (day 8), rats were anesthetized using diethyl ether before blood samples were carefully collected via cardiac puncture. All rats, without regaining consciousness, were sacrificed by overdose of diethyl ether.
Determination of serum NO and inflammatory cytokines
Blood samples were allowed to coagulate for 60 minutes in sterile blank tubes before being centrifuged at 3000 g for 15 minutes. Subsequently, the supernatant was maintained at -80 °C until commercially available nitrite/nitrate colorimetric kit analysis (Roche, Germany). The concentrations of nitrite and nitrate were measured at 540 nm using spectrophotometry (Infinte M200, Tecan, Austria).Using known amounts of potassium and sodium nitrate, a standard curve was created. The indicator of NO level employed was the sum of nitrite and nitrate. Using enzyme-linked immunosorbent assay (ELISA) kits, the levels of inflammatory cytokines (tumor necrosis factor (TNF)-α, interleukin (IL)-1β, and IL-10) in serum samples were measured in compliance with the manufacturer’s recommended methods (Thermo Scientific, US).
Determination of blood COX-1 and COX-2 enzymes
A similar procedure previously reported by Wallace26 was used to measure the COX-1 and COX-2 enzyme activity in blood samples. Blood was drawn into a blank glass tube and incubated at 37 °C for 45 minutes in order to measure COX-1 activity. After that, the samples were centrifuged for 15 minutes at 3000 g. Before being tested, the serum was moved to microcentrifuge tubes and kept at -80 °C. Under the conditions of this experiment, thromboxane B2 generated from COX-1 in platelet of blood samples was evaluated using a commercially available ELISA kit (Abnova, Taiwan). Each rat’s blood sample was split into two lithium heparin collection tubes with 10 µg/mL of acetylsalicylic acid to measure COX-2 activity. Additionally, 10 µg/mL of lipopolysaccharide from Escherichia coli, a bacterial endotoxin, was present in one of the tubes. Following a 24-hour incubation period at 37 °C in a shaking water bath, these tubes were centrifuged for 15 minutes at 3000 g. Aspirating the supernatant (plasma) samples into microcentrifuge tubes, they were kept at -80 °C until prostaglandin (PG) E2 was measured using a particular ELISA kit (Thermo Scientific, US). The quantity of PGE2 produced by the COX-2 enzyme was equal to the amount produced in the tubes containing endotoxin minus the amount in the other tube.
Statistical analyses
The analysis of variance was applied to the data, and then the groups were compared using Dunnet’s multiple comparison test and the two groups were compared using Student’s t-test. A significant P-value was defined as one that was less than 0.05, or P<0.05. SPSS 16.0 was used for all statistical analyses (SPSS Inc., US).
Results
Acetic acid-induced abdominal writhing test
Data obtained was stated in Table I and Figure 1. Both piroxicam and liposomes-encapsulated piroxicam exhibited dose-dependent pain inhibition effects at various experimental time points. Data showed that the highest inhibition of pain (≥70.19%) was shown in mice treated with 30 mg/kg liposome-encapsulated piroxicam. Lower doses of liposomes-encapsulated piroxicam (0.3 and 3 mg/kg) were also found to be adequate in producing statistically significant anti-nociceptive activities that lasted throughout duration of this test. Contrarily, only 3 and 30 mg/kg piroxicam produced a significant pain inhibitory activity that lasted for 10 and 30 minutes, respectively. Statistical comparisons among treatment groups with equivalent drug dosages successfully showed that liposome-encapsulated piroxicam formulations posed significantly higher antinociceptive effects than piroxicam at the dosage of 0.3, 3 and 30 mg/kg. The total percentage inhibition was increased by 20.15%, 18.02% and 26.66% at drug dosage of 0.3, 3 and 30 mg/kg, respectively.
![]() |
Figure 1: Number of abdominal writhing within 30 minutes (0-30th min). |
Table I: Number and percentage inhibition of abdominal writhing at different time intervals.
Treatment group |
Drug dosage (mg/kg) |
Number of abdominal writhing [Inhibition (%)] |
|||||
0-5th min |
5-10th min |
10-15th min |
15-20th min |
20-25th min |
25-30th min |
||
Piroxicam |
0 (Control) |
26.33 ±2.78 |
25.67 ±3.46 |
22.50 ±2.67 |
22.83 ±3.75 |
18.50 ±3.45 |
17.33 ±2.23 |
0.3 |
17.83 ±1.85 [32.28] |
18.83 ±1.91 [26.62] |
20.50 ±1.38 [8.89] |
17.67 ±1.74 [22.63] |
14.00 ±2.11 [24.32] |
13.83 ±2.04 [20.19] |
|
3 |
12.83 ±2.69* [51.27] |
16.00 ±1.29* [37.66] |
18.17 ±1.40 [19.26] |
17.83 ±3.44 [21.90] |
15.50 ±1.52 [16.22] |
11.50 ±1.34 [33.65] |
|
30 |
9.17 ±2.23* [65.19] |
12.00 ±2.05* [53.25] |
11.67 ±2.56* [48.15] |
8.67 ±1.56* [62.04] |
10.83 ±2.55 [41.44] |
7.00 ±1.34* [59.62] |
|
Liposomes-encapsulated piroxicam |
0 |
20.67 ±2.63 [21.52] |
18.00 ±2.07 [29.87] |
20.67 ±0.92 [8.15] |
15.83 ±1.35 [30.66] |
15.17 ±1.72 [18.02] |
14.33 ±1.87 [17.31] |
0.3 |
13.83 ±2.30* [47.47] |
17.17 ±1.96* [33.12] |
13.33 ±2.47*# [40.74] |
10.83 ±2.27*# [52.55] |
11.83 ±1.08 [36.04] |
8.83 ±1.52* [49.04] |
|
3 |
15.33 ±3.03* [41.77] |
15.17 ±1.47* [40.91] |
13.00 ±0.82*# [42.22] |
9.83 ±1.70* [56.93] |
9.00 ±2.00*# [51.35] |
5.50 ±1.31*# [68.27] |
|
30 |
3.33 ±1.05*# [87.34] |
4.00 ±1.44*# [84.42] |
4.50 ±0.92*# [80.00] |
2.83 ±1.14*# [87.59] |
4.00 ±0.89*# [78.38] |
5.17 ±0.95* [70.19] |
Values are mean ± S.E.M. (n=6/group)
*Significant difference (P<0.05) when compared to control at their respective time interval
#Significant difference (P<0.05) when compared to equivalent dosage of piroxicam at their respective time interval
Values in parenthesis are percentage of inhibition when compared to control at their respective time interval
Formalin-induced paw-licking test
Result obtained was shown in Table 2. Reduction of paw-licking time, which reflected an anti-nociceptive activity, was observed in dose-dependent manner during both early and late phases. Statistical analysis, however, showed that rats treated with 30 mg/kg of piroxicam and 3 and 30 mg/kg of liposome-encapsulated piroxicam only showed a significant reduction in the late phase (inflammatory phase) when compared to the control group. Their percentages of inflammatory pain inhibition were 62.88%, 47.46% and 81.36%, respectively. In addition, both 3 and 30 mg/kg liposomes-encapsulated piroxicam also showed significant greater paw-licking time reduction when compared to their equivalent dosages of piroxicam during late phase. Percentage inhibition was increased by 25.77% and 18.48% for drug dosage of 3 and 30 mg/kg, respectively.
Table 2: Paw-licking time and percentage inhibition at different phases.
Treatment group |
Drug dosage (mg/kg) |
Paw-licking time (s) [Inhibition (%)] |
|
Early phase |
Late phase |
||
Piroxicam |
0 (Control) |
89.83 ± 8.57 |
98.33 ± 9.38 |
0.3 |
82.00 ± 5.77 [8.72] |
82.50 ± 9.08 [16.10] |
|
3 |
76.83 ± 7.56 [14.47] |
77.00 ± 6.76 [21.69] |
|
30 |
75.33 ± 5.64 [16.14] |
36.50 ± 5.10* [62.88] |
|
Liposomes-encapsulated piroxicam |
0 |
87.50 ± 5.88 [2.60] |
90.67 ± 7.30 [7.80] |
0.3 |
71.83 ± 3.97 [20.04] |
73.17 ± 5.26 [25.59] |
|
3 |
67.00 ± 6.87 [25.42] |
51.67 ± 4.26*# [47.46] |
|
30 |
67.67 ± 6.43 [24.68] |
18.33 ± 6.32*# [81.36] |
Values are mean ± S.E.M. (n=6/group)
*Significant difference (P<0.05) when compared to control at their respective phase
#Significant difference (P<0.05) when compared to equivalent dosage of piroxicam at their respective phase
Values in parenthesis are percentage of inhibition when compared to control at their respective phase
Carrageenan-induced mechanical hyperalgesia test
The acquired data was displayed in Table 3. Rats treated with 30 mg/kg piroxicam and 3 and 30 mg/kg liposome-encapsulated piroxicam showed a considerably higher tolerance of mechanical hyperalgesia at different experimental time points than the control group, according to statistical analyses. However, 30 mg/kg liposome-encapsulated piroxicam was the only formulation with notable inhibitory effects that persisted throughout the entire test. Rats treated with 30 mg/kg liposomal piroxicam sample showed the two highest percentages of inhibition (118.7% and 120.9%) in the current investigation, which were discovered two and four hours after carrageenan injection. Interestingly, at these two time points, significant differences were also found when 30 mg/kg liposomes-encapsulated piroxicam was compared to 30 mg/kg piroxicam. The increment in percentages of inhibition was 44.9% and 55.8%, respectively.
Table 3: Paw pressure and percentage inhibition at different time intervals.
Treatment group |
Drug dosage (mg/kg) |
Pressure applied on paw (g) [Inhibition (%)] |
|||||
0h |
1h |
2h |
3h |
4h |
5h |
||
Piroxicam |
0 (Control) |
105.4 ±13.7 |
109.2 ±12.4 |
108.0 ±12.5 |
106.0 ±14.7 |
96.6 ±12.1 |
94.9 ±21.7 |
0.3 |
135.2 ±14.6 [28.2] |
120.4 ±18.2 [10.3] |
123.2 ±15.0 [14.1] |
124.8 ±17.4 [17.7] |
110.0 ±18.4 [13.9] |
110.2 ±15.5 [16.2] |
|
3 |
171.3 ±27.0 [62.5] |
153.0 ±19.4 [40.1] |
148.8 ±11.1 [37.8] |
151.0 ±19.6 [42.5] |
138.9 ±17.1 [43.8] |
127.8 ±14.4 [34.7] |
|
30 |
218.3 ±18.5* [107.1] |
209.0 ±14.6* [91.4] |
187.7 ±12.0* [73.8] |
194.7 ±17.3* [83.6] |
159.4 ±13.9 [65.1] |
151.1 ±15.0 [59.3] |
|
Liposomes-encapsulated piroxicam |
0 |
117.7 ±10.9 [11.6] |
117.7 ±22.3 [7.7] |
119.6 ±21.0 [10.7] |
121.3 ±21.0 [14.5] |
111.2 ±22.3 [15.2] |
110.0 ±15.7 [15.9] |
0.3 |
143.0 ±20.1 [35.6] |
146.7 ±19.4 [34.3] |
147.2 ±16.4 [36.3] |
143.3 ±16.7 [35.2] |
130.3 ±24.3 [35.0] |
127.3 ±21.7 [34.2] |
|
3 |
163.0 ±22.6 [54.6] |
176.7 ±17.3 [61.7] |
164.3 ±16.7 [52.2] |
178.3 ±15.9* [68.2] |
167.9 ±15.9* [73.9] |
157.3 ±25.4 [65.8] |
|
30 |
213.0 ±11.7* [102.0] |
220.8 ±19.1* [102.1] |
236.2 ±17.8*# [118.7] |
228.4 ±21.0* [115.5] |
213.3 ±14.0*# [120.9] |
199.1 ±19.5* [109.8] |
Values are mean ± S.E.M. (n=6/group)
*Significant difference (P<0.05) when compared to control at their respective time interval
#Significant difference (P<0.05) when compared to equivalent dosage of piroxicam at their respective time interval
Values in parenthesis are percentage of inhibition when compared to control at their respective time interval
Carrageenan-induced thermal hyperalgesia test
Result was shown in Table 4. Comparing to control group, significant prolongation of paw withdrawal latencies was observed during second hour after carrageenan injection in rats treated with 30 mg/kg piroxicam. At comparable experimental time periods, rats administered with 3 and 30 mg/kg liposome-encapsulated piroxicam likewise showed considerable inhibition when compared to the control group, with the highest percentage inhibition being 55.71%. Regretfully, when piroxicam and liposome-encapsulated piroxicam at equal dosages were statistically compared, there was no discernible difference in the inhibitions for thermal hyperalgesia.
Table 4: Latency time and percentage inhibition at different time intervals
Treatment group |
Drug dosage (mg/kg) |
Latency time of reaction (s) [Inhibition (%)] |
|||||
0h |
1h |
2h |
3h |
4h |
5h |
||
Piroxicam |
0 (Control) |
7.82 ±0.87 |
7.26 ±0.82 |
7.10 ±0.57 |
7.38 ±0.66 |
7.02 ±0.82 |
7.19 ±0.60 |
0.3 |
8.49 ±0.75 [8.67] |
8.97 ±0.64 [23.66] |
9.23 ±0.58 [30.05] |
9.23 ±0.67 [24.98] |
8.74 ±1.93 [24.62] |
8.38 ±1.59 [16.53] |
|
3 |
9.32 ±1.26 [19.19] |
9.33 ±1.25 [28.56] |
9.75 ±0.46 [37.32] |
9.55 ±1.30 [29.35] |
9.06 ±0.75 [29.14] |
9.45 ±1.87 [31.35] |
|
30 |
10.76 ±1.24 [37.67] |
10.42 ±0.93 [43.57] |
10.66 ±0.78* [50.08] |
10.46 ±0.60* [41.61] |
10.36 ±0.73 [47.59] |
10.82 ±0.83 [50.42] |
|
Liposomes-encapsulated piroxicam |
0 |
7.76 ±1.10 [-0.78] |
8.09 ±0.94 [11.56] |
7.97 ±0.57 [12.21] |
8.00 ±0.30 [8.35] |
7.79 ±0.56 [11.01] |
7.97 ±0.80 [10.81] |
0.3 |
9.08 ±0.74 [16.13] |
9.47 ±1.15 [30.47] |
9.04 ±1.05 [27.39] |
8.65 ±0.81 [17.16] |
9.03 ±1.92 [28.74] |
9.13 ±1.42 [26.87] |
|
3 |
9.38 ±1.14 [19.97] |
9.87 ±0.88 [35.99] |
10.13 ±0.80* [42.64] |
9.93 ±0.71 [34.54] |
10.03 ±0.64 [42.91] |
9.99 ±1.38 [38.92] |
|
30 |
10.59 ±1.23 [35.47] |
10.53 ±0.85 [45.18] |
11.06 ±0.67* [55.71] |
10.60 ±0.76* [43.57] |
10.71 ±0.77 [52.57] |
11.01 ±0.51 [52.97] |
Values are mean ± S.E.M. (n=6/group)
*Significant difference (P<0.05) when compared to control at their respective time interval
#Significant difference (P<0.05) when compared to equivalent dosage of piroxicam at their respective time interval
Values in parenthesis are percentage of inhibition when compared to control at their respective time interval
Brewer’s yeast-induced hyperthermia test
Data from anti-pyretic assay was presented in Table 5. Significantly lowered rectal temperatures comparing to control group were observed when the hyperthermic animals were orally treated with 30 mg/kg of piroxicam, both in non-encapsulated and liposomes-encapsulated forms. However, the former showed significant anti-hyperthermia effect at third hour following treatment whereas the latter posed its inhibitory activity after two hours. Treatment with 0.3 and 3 mg/kg of liposomes-encapsulated piroxicam also caused significant pyretic inhibition at a few experimental time points. The percentages of inhibitions were between 1.50% and 1.99%. However, no statistically significant difference between the piroxicam and liposome-encapsulated piroxicam at equivalent dosages at all tested time points.
Table 5: Rectal temperature and percentage inhibition at different time intervals.
Treatment group |
Drug dosage (mg/kg) |
Rectal temperature (°C) [Inhibition (%)] |
|||||
0h |
1h |
2h |
3h |
4h |
5h |
||
Piroxicam |
0 (Control) |
37.93 ±0.26 |
37.98 ±0.06 |
37.88 ±0.12 |
37.80 ±0.12 |
37.75 ±0.17 |
37.57 ±0.17 |
0.3 |
37.85 ±0.11 [0.22] |
37.73 ±0.10 [0.66] |
37.60 ±0.11 [0.75] |
37.53 ±0.13 [0.71] |
37.35 ±0.11 [1.06] |
37.27 ±0.16 [0.80] |
|
3 |
37.97 ±0.18 [-0.09] |
37.68 ±0.13 [0.79] |
37.47 ±0.21 [1.10] |
37.53 ±0.26 [0.71] |
37.40 ±0.16 [0.93] |
37.20 ±0.26 [0.98] |
|
30 |
37.98 ±0.26 [-0.13] |
37.48 ±0.20 [1.32] |
37.30 ±0.20 [1.54] |
36.97 ±0.19* [2.20] |
36.88 ±0.09* [2.30] |
36.88 ±0.13* [1.82] |
|
Liposomes-encapsulated piroxicam |
0 |
37.93 ±0.12 [0.00] |
37.83 ±0.21 [0.39] |
37.80 ±0.14 [0.22] |
37.78 ±0.10 [0.04] |
37.52 ±0.16 [0.62] |
37.47 ±0.09 [0.27] |
0.3 |
37.92 ±0.05 [0.04] |
37.70 ±0.15 [0.75] |
37.60 ±0.14 [0.75] |
37.27 ±0.20 [1.41] |
37.18 ±0.21* [1.50] |
37.07 ±0.19 [1.33] |
|
3 |
37.80 ±0.14 [0.35] |
37.57 ±0.08 [1.10] |
37.27 ±0.14* [1.63] |
37.13 ±0.12* [1.76] |
37.00 ±0.14* [1.99] |
36.95 ±0.06* [1.64] |
|
30 |
37.90 ±0.12 [0.09] |
37.32 ±0.11* [1.76] |
37.23 ±0.16* [1.72] |
36.85 ±0.11* [2.51] |
36.75 ±0.08* [2.65] |
36.73 ±0.08* [2.22] |
Values are mean ± S.E.M. (n=6/group)
*Significant difference (P<0.05) when compared to control at their respective time interval
#Significant difference (P<0.05) when compared to equivalent dosage of piroxicam at their respective time interval
Values in parenthesis are percentage of inhibition when compared to control at their respective time interval.
Carrageenan-induced paw-edema test
Result obtained was showed in Table 6. Both piroxicam and liposomes-encapsulated piroxicam samples at 0.3, 3 and 30 mg/kg exhibited significant anti-inflammatory effects as compared to control group at various time points following carrageenan injection. Nevertheless, in contrast to the piroxicam, 0.3 and 3 mg/kg liposomes-encapsulated piroxicam were found to demonstrate significant paw-edema inhibitory activities that lasted until the final experimental time point (fifth hour) in present study. Further statistical analyses revealed that the 3 and 30 mg/kg liposomes-encapsulated piroxicam successfully resulted in a significant decrement of paw-edema volumes when compared to their equivalent piroxicam dosage groups after the fifth and third hour, respectively. In term of percentages of inhibition, an increment between 23.81% and 28.57% was observed during this time frame.
Table 6: Edema volume and percentage inhibition at different time intervals
Treatment group |
Drug dosage (mg/kg) |
Edema volume (mL) [Inhibition (%)] |
||||
1h |
2h |
3h |
4h |
5h |
||
Piroxicam |
0 (Control) |
0.48 ±0.07 |
0.43 ±0.09 |
0.55 ±0.09 |
0.50 ±0.11 |
0.50 ±0.08 |
0.3 |
0.29 ±0.05* [40.00] |
0.21 ±0.03 [50.00] |
0.33 ±0.05 [39.13] |
0.24 ±0.05* [52.38] |
0.29 ±0.06 [42.86] |
|
3 |
0.29 ±0.04* [40.00] |
0.26 ±0.07 [38.89] |
0.26 ±0.06* [52.17] |
0.24 ±0.05* [52.38] |
0.26 ±0.04 [47.62] |
|
30 |
0.29 ±0.05* [40.00] |
0.17 ±0.06* [61.11] |
0.21 ±0.03* [60.87] |
0.24 ±0.03* [52.38] |
0.17 ±0.04* [66.67] |
|
Liposomes-encapsulated piroxicam |
0 |
0.48 ±0.05 [0.00] |
0.36 ±0.08 [16.67] |
0.45 ±0.09 [17.39] |
0.38 ±0.05 [23.81] |
0.38 ±0.11 [23.81] |
0.3 |
0.24 ±0.03* [50.00] |
0.29 ±0.05 [33.33] |
0.26 ±0.04* [52.17] |
0.26 ±0.04* [47.62] |
0.24 ±0.05* [52.38] |
|
3 |
0.19 ±0.03* [60.00] |
0.21 ±0.07 [50.00] |
0.29 ±0.04* [47.83] |
0.21 ±0.05* [57.14] |
0.12 ±0.04*# [76.19] |
|
30 |
0.17 ±0.02* [65.00] |
0.10 ±0.03* [77.78] |
0.07 ±0.05*# [86.96] |
0.12 ±0.02*# [76.19] |
0.05 ±0.03*# [90.48] |
Values are mean ± S.E.M. (n=6/group)
*Significant difference (P<0.05) when compared to control at their respective time interval
#Significant difference (P<0.05) when compared to equivalent dosage of piroxicam at their respective time interval
Values in parenthesis are percentage of inhibition when compared to control at their respective time interval
Cotton pellet-induced granuloma test
Results (Table 7) showed a dose-dependent chronic anti-inflammatory effect by piroxicam and liposomes-encapsulated piroxicam. Data obtained showed that treatment using liposomes-encapsulated piroxicam at 3 and 30 mg/kg significantly reduced both the transudative and granuloma weights by more than 36.41%. On the contrary, only highest dose (30 mg/kg) of non-encapsulated piroxicam resulted in statistically significant inhibitory activities as compared to control group. The percentage inhibition for transudative and granuloma weights were only 30.97% and 32.89%, respectively. Further statistical analyses proved that liposomal piroxicam samples possessed greater anti-transudative and anti-granuloma effects when compared to piroxicam of same dosage. Significantly different in transudative weight was found between piroxicam and liposomes-encapsulated piroxicam at 3 mg/kg. The transudative weight was 18.3% higher when piroxicam was used to treat the animals in present experiment. In addition, 3 and 30 mg/kg liposomes-encapsulated piroxicam samples respectively resulted in 33.31% and 18.90% greater inhibition of granuloma weight than the piroxicam samples of equivalent dosage.
Table 8: Transudative weight, granuloma weights and percentage inhibition.
Treatment group |
Drug dosage (mg/kg) |
Weight (mg) [Inhibition (%)] |
|
Transudative |
Granuloma |
||
Piroxicam |
0 (Control) |
429.90 ± 47.52 |
90.82 ± 11.97 |
0.3 |
376.95 ± 37.44 [12.32] |
78.92 ± 12.37 [13.10] |
|
3 |
352.05 ± 19.22 [18.11] |
76.33 ± 3.75 [15.95] |
|
30 |
296.75 ± 22.48* [30.97] |
60.95 ± 5.59* [32.89] |
|
Liposomes-encapsulated piroxicam |
0 |
428.10 ± 22.87 [0.42] |
82.32 ± 5.90 [9.36] |
0.3 |
353.03 ± 19.35 [17.88] |
68.98 ± 3.51 [24.04] |
|
3 |
273.37 ± 20.95*# [36.41] |
46.08 ± 7.07*# [49.26] |
|
30 |
243.92 ± 19.60* [43.26] |
43.78 ± 5.08*# [51.79] |
Values are mean ± S.E.M. (n=6/group)
*Significant difference (P<0.05) when compared to control
#Significant difference (P<0.05) when compared to equivalent dosage of piroxicam
Values in parenthesis are percentage of inhibition when compared to control
NO activity
The data obtained (Table 8) demonstrated that, in comparison to the control group, repeated treatment of either piroxicam or liposome-encapsulated piroxicam at the highest dose of 30 mg/kg resulted in a considerable reduction of NO. In comparison to the control, a lower dosage of liposome-encapsulated piroxicam (3 mg/kg) was sufficient to cause a considerable reduction in serum NO levels. Subsequent statistical analysis showed that the piroxicam encapsulated in liposomes at a dose of 30 mg/kg significantly inhibited NO more than the piroxicam at the same dosage. It was discovered to have an 18.72% higher percentage of inhibition than the piroxicam sample.
Table 8: Effects of different treatment upon serum NO.
Treatment group |
Drug dosage (mg/kg) |
Concentration (µM) [Inhibition (%)] |
Piroxicam |
0 (Control) |
18.96 ± 0.97 |
0.3 |
17.52 ± 1.36 [7.59] |
|
3 |
16.08 ± 1.51 [15.17] |
|
30 |
11.58 ± 1.23* [38.91] |
|
Liposomes-encapsulated piroxicam |
0 |
17.08 ± 1.30 [9.90] |
0.3 |
14.23 ± 1.65 [24.94] |
|
3 |
12.14 ± 1.62* [35.98] |
|
30 |
8.03 ± 0.95*# [57.63] |
Values are mean ± S.E.M. (n=6/group)
*Significant difference (P<0.05) when compared to control
#Significant difference (P<0.05) when compared to equivalent dosage of piroxicam
Values in parenthesis are percentage of inhibition when compared to control
Inflammatory cytokines activity
Data obtained was summarized in Table IX. Analyses showed that piroxicam only resulted in a significant change to serum TNF-α at the highest dose (30 mg/kg). Whereas a lower dose (0.3 or 3 mg/kg) of liposomes-encapsulated piroxicam were adequate to cause statistically significant TNF-α and IL-1β inhibition. Further analyses revealed that 3 and 30 mg/kg liposomes-encapsulated piroxicam samples possessed significantly greater inhibition of TNF-α and IL-1β respectively than the effects exhibited by non-encapsulated piroxicam of equivalent dosages. Contrarily, only the 30 mg/kg liposomes-encapsulated piroxicam sample successfully resulted in significant increment of serum IL-10 when compared to control group. There was no statistical difference found between the liposome-encapsulated piroxicam and equivalent dose of piroxicam for serum IL-10.
Table 9: Effects of different treatment upon serum TNF-α, IL-1β and IL-10
Treatment group |
Drug dosage (mg/kg) |
Concentration (pg/mL) [Inhibition (%)] |
||
TNF-α |
IL-1β |
IL-10 |
||
Piroxicam |
0 (Control) |
55.80 ± 6.38 |
56.88 ± 6.77 |
46.18 ± 3.47 |
0.3 |
44.27 ± 4.91 [20.66] |
46.58 ± 6.08 [18.11] |
54.76 ± 5.16 [18.57] |
|
3 |
45.44 ± 4.04 [18.57] |
39.00 ± 4.03 [31.44] |
50.92 ± 7.15 [10.26] |
|
30 |
32.90 ± 4.80* [41.03] |
43.26 ± 3.27 [23.95] |
60.47 ± 4.85 [30.93] |
|
Liposomes-encapsulated piroxicam |
0 |
53.43 ± 3.89 [4.25] |
53.66 ± 6.62 [5.67] |
50.35 ± 5.02 [9.03] |
0.3 |
44.51± 6.93 [20.23] |
34.18 ± 5.59* [39.91] |
52.34 ± 3.20 [13.34] |
|
3 |
32.55 ± 3.92*# [41.66] |
35.76 ± 4.60* [37.14] |
61.46 ± 4.05 [33.09] |
|
30 |
22.85 ± 4.26* [59.05] |
29.63 ± 4.56*# [47.92] |
65.21± 4.32* [41.20] |
Values are mean ± S.E.M. (n=6/group)
*Significant difference (P<0.05) when compared to control
#Significant difference (P<0.05) when compared to equivalent dosage of piroxicam
Values in parenthesis are percentage of inhibition when compared to control
Blood COX-1 and COX-2 activities
The dose-dependent inhibition of COX enzymes by different treatment samples was summarized in Table 10. Results showed that both piroxicam and liposomes-encapsulated piroxicam significantly reduced serum concentration of COX-1 enzyme only at the highest drug dosage of 30 mg/kg. Further analyses demonstrated no significant difference between the two treatment groups. In contrast, rats which were treated with piroxicam at 3 and 30 mg/kg as well as liposomes-encapsulated piroxicam at 0.3, 3 and 30 mg/kg significantly inhibited the production of COX-2 enzyme. Statistical comparisons between treatment groups with equivalent drug dosage successfully showed that liposomes-encapsulated piroxicam (3 and 30 mg/kg) posed significantly greater COX-2 enzyme inhibition than piroxicam in non-encapsulated form. The total percentage inhibition was increased by 18.25% and 19.22% at drug dosage of 3 and 30 mg/kg, respectively.
Table X: Effects of different treatment upon blood COX-1 and COX-2
Treatment group |
Drug dosage (mg/kg) |
Concentration (ng/mL) [Inhibition (%)] |
|
COX-1 |
COX-2 |
||
Piroxicam |
0 (Control) |
41.04 ± 4.15 |
25.40 ± 2.24 |
0.3 |
37.33 ± 5.58 [9.04] |
21.83 ± 1.81 [14.05] |
|
3 |
26.39 ± 2.97 [35.70] |
16.48 ± 1.43* [35.14] |
|
30 |
17.99 ± 3.31* [56.16] |
11.87 ± 1.70* [53.29] |
|
Liposomes-encapsulated piroxicam |
0 |
40.12 ± 5.65 [2.25] |
24.01 ± 1.70 [5.49] |
0.3 |
36.16 ± 4.52 [11.88] |
17.77 ± 2.57* [30.03] |
|
3 |
28.65 ± 4.76 [30.18] |
11.84 ± 1.11*# [53.39] |
|
30 |
17.64 ± 3.67* [57.02] |
6.98 ± 0.76*# [72.51] |
Values are mean ± S.E.M. (n=6/group)
*Significant difference (P<0.05) when compared to control
#Significant difference (P<0.05) when compared to equivalent dosage of piroxicam
Values in parenthesis are percentage of inhibition when compared to control
Discussion
Fever, inflammation, and pain have all been linked to the pathophysiology of a number of clinical disorders, including cancer, vascular illnesses, and arthritis27, 28. As a result, it is thought to be crucial for the liposomal delivery method to enhance the in vivo therapeutic activities (anti-nociceptive, anti-pyretic, and anti-inflammatory effects) of piroxicam, especially in order to better manage the symptoms and indicators of these illnesses. In present study, several animal models were employed to evaluate the effectiveness of present liposomal formulations in improving in vivo therapeutic activities of orally administered piroxicam at three different dose levels.
It is generally known that, in animal models, the assessment of pain perception can be done by examining overt behavioral reactions or nociceptive reflexes that are directly induced by noxious stimuli (such as chemical, thermal, electrical, and mechanical ones).29, 30. An very sensitive assay that is frequently used to screen drugs for anti-nociceptive activity at dose levels that may look inert in other approaches is the acetic acid-induced abdominal writhing test25, 31. Intraperitoneal injection of acetic acid, which irritates serous membranes and produce peritoneal inflammation, provokes a very stereotyped behavior known as writhing effect32. Administration of relatively small doses of NSAIDs or opioids is known to abolish the writhing response in a dose dependent manner33, 34. The present study’s data demonstrated that piroxicam and piroxicam formulations encapsulated in liposomes both demonstrated dose-dependent pain inhibitory properties, as evidenced by a decrease in the number of writhing effects. The results of this chemonociception test also showed that, in comparison to piroxicam at equal dosages, piroxicam encapsulated in liposomes demonstrated a more potent and sustained anti-nociceptive effect. However, liposome encapsulation has several limitations such as poor molecular targeting, instability, short circulation time in vivo and low encapsulation efficiency1,3. Interestingly, we have demonstrated the solution of these limitation of piroxicam encapsulation previously18,19.
The acetic acid-induced abdominal writhing test, however, shows poor specificity as it does not indicate whether an anti-nociceptive activity is central and/or peripheral29, 35. Acetic acid itself may cause pain, while at the same time stimulates peripheral tissues to release endogenous substances such as serotonin, histamine, PG (e.g. PGE2, PGF2α), bradykinin and substance P that sensitize pain nerve endings (nociceptors) which in turn causes pain at the location36, 37. The anti-nociception activity of a compound may therefore be due to its action on visceral receptors that are sensitive to acetic acid, the inhibition of algogenic substances production or the inhibition of painful messages transmission at central level31. Therefore, the formalin-induced paw licking test was carried out to strengthen further evidences of improved anti-nociceptive activity that was observed in writhing test.
A valid model of tonic pain that mimics clinical pain circumstances in humans is the formalin-induced paw licking test. This assay has a solid track record of helping to clarify a compound’s mode of action24,38, 39. Formalin injected intraplantarly into the paw causes two separate phases, each of which represents a different kind of pain. The early phase, which represents centrally mediated pain (neurogenic pain), is a direct result of stimulation of nociceptors in the paw. On the other hand, the late phase is brought on by inflammation, which causes nociceptors to become sensitized and produce pain (inflammatory pain) when algogenic substances are released from damaged tissues. There is also, at least to some degree, sensitization of central nociceptive neurons during late phase25, 34. Thus, drugs that act primarily on central nervous system (e.g. opiods) are known to inhibit both phases equally, whereas peripherally acting drugs (e.g. NSAIDs) are more effective in inhibiting the late phase33, 35. Data in present study revealed that liposomes-encapsulated piroxicam possessed significantly greater nociception inhibition than piroxicam during the late phase only. This finding suggested that liposomes-encapsulated piroxicam resulted in a more effective suppression of inflammatory pain, hence supported the fact that a stronger peripherally mediated anti-nociceptive activity could be attained using the present liposomal formulations.
One ubiquitous issue that frequently causes both spontaneous pain and hyperalgesia is inflammatory pain. After cutaneous injury and/or inflammation, the hyperalgesia response (heightened sensitivity to pain) is defined by a peripheral sensitization of nociceptors due to an increase in neuronal membrane excitability to inflammatory mediators30. The potential advantages of liposomal formulations in lowering mechanical and thermally induced hyperalgesia responses were assessed in the current investigation. In animal models, carrageenan, a family of linear sulphated polysaccharides isolated from the marine red seaweed Chondrus crispu, was utilized to cause transient inflammation and hyperalgesia40. Because carrageenan-induced inflammatory models can closely mimic various real pain syndromes, they have been widely used in the field of pain research41. The results of the current hyperalgesia experiments demonstrated that piroxicam encapsulated in liposomes may raise animals’ nociceptive thresholds to heat and mechanical stimuli at both highest dosage levels, indicating that lower drug dosages may be necessary to achieve anti-hyperalgesia benefits. In addition, statistical comparisons between treatment groups with equivalent drug dosages revealed that animal’s tolerance to mechanical-induced hyperalgesia response could also be increased using present liposomal formulations.
Fever is resulted during tissue damage, inflammation, infection or disease states. A pathogenic fever can be induced in animal model by administration of yeast. Under these conditions, formation of cytokines and thus the synthesis of PG are increased 32. NSAIDs exert their anti-pyretic effect by inhibition of PGE2 synthesis, which is responsible for triggering hypothalamus to increase body temperature42. Data obtained in present study showed that liposomal formulation was able to result in a faster onset of anti-pyretic effect, even though the intensity of effect was not significantly increased. Moreover, in comparison to non-encapsulated form of piroxicam, lower dosages of liposomes-encapsulated piroxicam were found to be sufficient in producing significant anti-pyretic effects.
The effects of the liposomal drug encapsulation strategy on the anti-inflammatory properties of piroxicam were assessed in addition to its anti-nociceptive and anti-pyretic properties. An indication of acute inflammatory alterations was a very reproducible edema that formed after an intraplantar injection of carrageenan42. In the current investigation, the first- and third-hours following carrageenan administration were when inflammatory edema peaked. It is widely established that paw edema caused by carrageenan is a biphasic event: PG and lysosome enzymes are released two to three hours after carrageenan injection, while serotonin, histamine, and kinins are released during the initial phase (first hour)31. Data obtained in present carrageenan-induced paw edema test indicated that, at two highest dose levels, liposomes-encapsulated piroxicam exhibited stronger acute anti-inflammatory activity than piroxicam. A higher inhibition of paw edema during the second phase suggested that piroxicam might attenuate release of PG or lysosome enzymes more effectively when the drug was formulated using liposomes.
On the other hand, chronic inflammation results from an initial response that is unable to completely remove proinflammatory chemicals, leading to neutrophil infiltration and exudation as well as fibroblast proliferation43. A common method for determining how well a substance inhibits the transudative and proliferative aspects of chronic inflammation is the cotton pellet-induced granuloma test44. By regulating mucopolysaccharides, decreasing collagen fiber synthesis, and limiting granulocyte infiltration to foreign implanted bodies, NSAIDs can reduce the growth of granuloma tissue, which is the outcome of a cellular reaction. However, it is reported that the inhibitory activities exerted by NSAIDs are only slight, whereas steroidal anti-inflammatory agents can strongly inhibit both transudative and proliferative phases25. Data from present study proved that the present liposomal formulations were able to increase anti-transudative and anti-granuloma effects of piroxicam.
All NSAIDs, including piroxicam, are known to exhibit their therapeutic activities through inhibition of various mediators, which in turn result in blockage of pathways that contribute to pathologic conditions such as pain, fever and inflammation44. As an attempt to gain further insights into the molecular basis of underlying mechanisms that contributed to the improved in vivo therapeutic activities of piroxicam using present liposomal formulations, the production of different key inflammatory mediators in animal was evaluated. Blood samples from rats in cotton pellet-induced granuloma test were used for present evaluation since a model of chronic inflammation involved a complex response which released numerous inflammatory mediators and resulted in multiple interactions39.
NO is a short-lived regulatory molecule which mediates diverse physiological processes such as vasodilatation and neurotransmission. While NO serves as a vital, multipurpose chemical messenger in biological systems, inducible NOS is known to produce excessive amounts of NO, which is linked to multiple forms of inflammation and multistage carcinogenesis in inflammatory sites46. Considerable evidence has demonstrated that NO and its synthases play pivotal roles in development and maintenance of edema, pain and hyperalgesia during inflammation 2,19. Besides the NO pathway, it is also well-established that a distinct cytokines cascade that unfolds during inflammatory processes can contribute to the development of inflammatory pain and hyperalgesia. The increased production of TNF-α during inflammation stimulates expression of other proinflammatory mediators (e.g. IL-1β, IL-6 and granulocyte-macrophage colony-stimulating factors), facilitates inflammatory cell infiltration and promotes induction of COX enzymes (39). Both TNF-α and IL-1β are reported to contribute to a hyperalgesia state through activation or sensitization of peripheral nociceptors, thus decreasing threshold of nociceptor during inflammation47. However, anti-inflammatory cytokines (such IL-10) that are released by monocytes and lymphocytes can prevent the generation of proinflammatory cytokines, which in turn causes an anti-inflammatory effect in the test model48.
Additionally, the level of PG is increased during an inflammatory process. PG can intensify and extend the signals generated by other molecular messengers, such as NO and proinflammatory cytokines, even when they do not cause inflammation on their own48. It has been demonstrated that the inhibition of PG production by NSAIDs effectively reduces inflammatory symptoms such as pain and edema49. The primary enzyme responsible for converting arachidonic acids into PG is COX. COX comes in two different isoforms: COX-1 and COX-2. It is well established that COX-1 supplies PG at a physiological level necessary for normal kidney, stomach, and platelet function; in contrast, COX-2 has been shown to be strongly stimulated by proinflammatory mediators, which raises PG synthesis during inflammation50. While it has been proposed that constitutively expressed COX-1 contributes to inflammatory processes, it is generally acknowledged that reduced COX-2 synthesis or activity oversees anti-inflammatory effects in both localized and systemic settings37. NSAIDs inhibit both COX isoenzymes, but to different degrees7.
The current investigation found that the suppression of COX-1 in the animal model was unaffected by liposomal formulation. On the other hand, at equivalent dosages, piroxicam encapsulated in liposomes demonstrated a higher degree of COX-2 inhibition than piroxicam. Additionally, it has been shown that at lesser dosages, piroxicam encapsulated in liposomes effectively suppresses COX-2. Therefore, it can be hypothesized that current liposomal formulations enhanced the piroxicam’s therapeutic effects by selectively inhibiting COX-2.
Conclusion
In summary, present study successfully revealed that the liposomal drug formulations improved in vivo therapeutic activities for piroxicam. As compared with non-encapsulated form of piroxicam, the liposomal piroxicam formulations were shown to exhibit stronger and longer-lasting peripherally mediated anti-nociceptive effects in animal models. Lower dosages of liposome-encapsulated piroxicam may result in a considerable suppression of acetic acid-induced abdominal writhing, formalin-induced paw licking, and carrageenan-induced mechanical and thermal hyperalgesia, according to results from the nociception assays. Besides enhancing latency threshold for inflammatory pain, lower dosage of liposomes-encapsulated piroxicam was also found to be enough in resulting anti-pyretic effect in Brewer’s yeast-induced hyperthermia test. Furthermore, even when lower medication dosages were employed to treat animals, the current investigation demonstrated that piroxicam encapsulated in liposomes held much stronger acute and chronic anti-inflammatory properties than piroxicam. The improved in vivo therapeutic activities by present liposomal delivery system were probably mediated via enhanced inhibition of proinflammatory mediators including NO, TNF-α and IL-1β. Besides, liposomal formulations were also shown to modulate the release of IL-10, an anti-inflammatory cytokine. Moreover, present liposomal formulation also resulted in an effective down-regulation of COX-2 but not COX-1 mediated PG synthesis in animal. Thus, these may prove very useful in clinical settings. Regardless of these excellent results, future studies are needed to fully understand the exact mechanism and extensive toxicological assessment for safety.
Acknowledgment
The authors thank Animal Care and Use Committee of Faculty of Medicine and Health Sciences, Universiti Putra Malaysia.
Conflict of Interest
There is no conflict of interest
Funding Source
This research was supported by Fundamental Research Grant Scheme from the Ministry of Higher Education, Malaysia (04-10-07-277FR).
References
- Ertekin Z.C, Bayindir Z.S and Yuksel N. Stability studies on piroxicam encapsulated niosomes. Drug Development and Industrial Pharmacy Current Drug Delivery 2015, 12(2), 192–199
CrossRef - El Sharkasy M.E, Tolba M.M, Belal F, Walash M.I and Aboshabana, R. Thiosemicarbazide functionalized carbon quantum dots as a fluorescent probe for the determination of some oxicams: application to dosage forms and biological fluids. RSC Advances 2021, 12(22), 13826–13836
CrossRef - Ambrogi V, Perioli L, Marmottini F, Giovagnoli S, Esposito M and Rossi C. Improvement of dissolution rate of piroxicam by inclusion into MCM-41 mesoporous silicate. Eu J Pharmaceutical Sc 2007;32(3):216-222.
CrossRef - Prabhu S, Ortega M and Ma C. Novel lipid-based formulations enhancing the in vitro dissolution and permeability characteristics of a poorly water-soluble model drug, piroxicam. Int J Pharmaceutics 2005;301(1-2):209-216.
CrossRef - Schäufele T.J, Kolbinger A, Friedel J, Pierre S and Scholich, K. Meloxicam treatment disrupts the regional structure of innate inflammation sites by targeting the pro-inflammatory effects of prostanoids. Bri J Pharmacol. 2024, 181(7), 1051–1067
CrossRef - Gwak H-S, Choi J-S and Choi H-K. Enhanced bioavailability of piroxicam via salt formation with ethanolamines. Int J Pharmaceutics 2005;297(1-2):156-161.
CrossRef - Oronsky B. INFLAMMASOME INHIBITORS 21st Century Miracle Drugs: Spotlight on Clinical NLRP3 Inflammasome Inhibitors. Drug Development and Delivery 2023, 23(3), 58–62
- Karatas A, Yüksel N and Baykara T. Improved solubility and dissolution rate of piroxicam using gelucire 44/14 and labrasol. Il Farmaco 2005;60(9):777-782.
CrossRef - Mosby. Piroxicam. In. 2007 Mosby’s Drug Consult. 17th edition, revised. St. Louis: Elsevier – Health Sciences Division; 2006. p.II 2336-II 2338.
- Rasetti-Escargueil C and Grange V. Pharmacokinetic profiles of two tablet formulations of piroxicam. Int J Pharmaceutics 2005;295(1-2):129-134.
CrossRef - Tagliati C.A, Kimura E, Nothenberg M.S, Santos S.R.J.C and Oga S. Pharmacokinetic profile and adverse gastric effect of zinc-piroxicam in rats. General Pharmacol: The Vascular System 1999;33(1):67-71.
CrossRef - MacLachlan I. Liposomal formulations for nucleic acid delivery. In: Crooke ST, editor. Antisense drug technology: principles, strategies, and applications. Boca Raton: CRC Press; 2007. p.237-270.
CrossRef - Chakraborty S, Shukla D, Mishra B and Singh S. Lipid – An emerging platform for oral delivery of drugs with poor bioavailability. Eu J Pharmaceutics and Biopharmaceutics 2009;73(1):1-15.
CrossRef - Kazi M., Alqahtani A., Ahmad A., Alqahtani A.S and Alanazi, FK. Development and optimization of sitagliptin and dapagliflozin loaded oral self-nanoemulsifying formulation against type 2 diabetes mellitus. Drug Delivery 2021, 28(1), 100–114
CrossRef - Fricker G, Kromp T, Wendel A, Blume A, Zirkel J, Rebmann H, Setzer C, Quinkert R-O, Martin F and Goymann CM. Phospholipids and lipid-based formulations in oral drug delivery. Pharmaceutical Research 2010;27(8):1469-1486.
CrossRef - Li X, Peng X, Zoulikha M, Ju Y and He, W. Multifunctional nanoparticle-mediated combining therapy for human diseases: an update review. Signal Transduction and Targeted Therapy 2024, 9(1), 1
CrossRef - Lucchetti G, Assogna G, Bagnato P and Janssen Farmaceutici S.A. Liposomal piroxicam formulations. United States Patent 5505960. 1996 April 9.
- Chiong H.S, Yong Y.K, Ahmad Z., Sulaiman M.R., Zakaria Z.A., Yuen K.H and Hakim M.N. Cytoprotective and enhanced anti-inflammatory activities of liposomal piroxicam formulation in lipopolysaccharide-stimulated RAW 264.7 macrophages. Int J Nanomed. 2013;2013(8):1245-1255.
CrossRef - Chiong H.S., Nazrul Hakim M., Sulaiman M.R., Zakaria Z.A., Zuraini A., Ong SGM and Yuen KH. Development and characterisation study of liposomes-encapsulated piroxicam. Int J Drug Delivery 2011;3(1):64-73.
CrossRef - Sulaiman M.R., Zakaria Z.A., Chiong H.S., Lai S.K., Israf D.A and Shah TMTA. Antinociceptive and anti-inflammatory effects of Stachytarpheta jamaicensis (L.) Vahl (Verbenaceae) in experimental animal models. Med Principles Practice 2009;18(4):272-279.
CrossRef - Mossadeq W.M.S, Sulaiman M.R., Mohamad T.A.T., Chiong H.S., Zakaria Z.A., Jabit M.L., Baharuldin M.T.H and Israf DA. Anti-inflammatory and antinociceptive effects of Mitragyna speciosa Korth methanolic extract. Med Principles Practice 2009;18(5):378-384.
CrossRef - Mizumura K, Taguchi T. Delayed onset muscle soreness: Involvement of neurotrophic factors. Journal of Physiological Sciences. 2016; 66: 43-52.
CrossRef - Ortiz MI, Ponce-Monter H, Fernández-Martínez E, Pérez-Hernández N, Macías A, Rangel-Flores E, Castañeda-Hernández G. Evaluation of the interaction between acemetacin and opioids on the hargreaves model of thermal hyperalgesia. Pharmacology, Biochemistry and Behavior 2007;88(1):47-54.
CrossRef - Yong Y.K., Somchit M.N and Ahmad Z. Anti-inflammatory properties of Bixa Orellana leaves extract are associated with suppression of bradykinin-induced endothelial hyperpermeability Pharmacognosy Magazine 2018, 14(57), S352–S357
CrossRef - Somchit M.N, Mohamed N.A, Ahmad Z, Fauzee M.S.O and Kadir AA. Anti-inflammatory and anti-pyretic properties of Spirulina platensis and Spirulina lonar: A comparative study. Pak J Pharmaceutical Sc 2014, 27(5), 1277–1280
CrossRef - Ferrer MD, Busquets-Cortés C, Capó X, Tejada S, Tur JA, Pons A, Sureda A. Cyclooxygenase-2 Inhibitors as a Therapeutic Target in Inflammatory Diseases. Current Medicinal Chemistry. 2019;26(18):3225-3241.
CrossRef - Raduan S.Z., Abdul Aziz M.W.H., Roslida A.H., Zuraini A and Hakim, MN. Anti- inflammatory effects of Hibiscus rosa-sinensis L. and Hibiscus rosa-sinensis var. alba ethanol extracts. Int J Pharmacy and Pharmaceutical Sciences 2013, 5(4), 754–762
- Somchit MN, Shukriyah MHN, Bustamam AA, Zuraini A. Anti-pyretic and analgesic activity of Zingiber zerumbet. Int J Pharmacology 2005;1(3):277-280.
CrossRef - Bars DL, Gozariu M and Cadden SW. Animal model of nociception. Pharmacological Rev 2001;53(4):597-652.
- Lavich TR, Cordeiro RSB, Silva PMR and Martins MA. A novel hot-plate test sensitive to hyperalgesic stimuli and non-opioid analgesics. Brazilian J Medical and Biological Research 2005;38(3):445-451.
CrossRef - Yerima M, Magaji MG, Yaro AH, Tanko Y and Mohammed MM. Analgesic and anti-inflammatory activities of the methanolic leaves extract of Securinega virosa (Euphorbiaceae). Nigerian J Pharmaceutical Sci 2009;8(1):47-53.
- Birari R.B., Jalapure S.S., Changrani S.R., Shid S.L., Tote M.V and Habade BM. Antiinflammatory, analgesic and antipyretic effect of Hibiscus Rosa Sinesis Lin flower. Pharmacologyonline 2009;3(1):737-747.
- Parker A.G., Peraza G.G., Sena J., Silva ES., Soares M.C.F., Vaz M.R.C., Furlong E.B and Muccillo-Baisch AL. Antinociceptive effects of the aqueous extract of Brugmansia suaveolens flowers in mice. Biological Research For Nursing 2007;8(3):234-239.
CrossRef - Vongtau HO, Abbah J, Ngazal IE, Kunle OF, Chindo BA, Otsapa PB, Gamaniel KS. Anti-nociceptive and anti-inflammatory activities of the methanolic extract of Parinari polyandra stem bark in rats and mice. Journal of Ethnopharmacology 2004;90(1):115-121.
CrossRef - Magaji MG, Anuka JA, Abdu-Aguye I, Yaro AH and Hussaini IM. Preliminary studies on anti-inflammatory and analgesic activities of Securinega virosa (Euphorbiaceae) in experimental animal models. J Medicinal Plants Res 2008;2(2):39-44.
- Intahphuak S, Panthong A, Kanjanapothi D, Taesotikul T, Krachangchaeng C and Reutrakul V. Anti-inflammatory and analgesic activities of Mallotus spodocarpus Airy Shaw. J Ethnopharmacol 2004;90(1):69-72.
CrossRef - Shu X-S, Gao Z-H and Yang X-L. Anti-inflammatory and anti-nociceptive activities of Smilax china L. aqueous extract. J Ethnopharmacol 2006;103(3):327-332.
CrossRef - Daud A., Habib N and Riera AS. Anti-inflammatory, anti-nociceptive and antipyretic effects of extracts of Phrygilanthus acutifolius flowers. J Ethnopharmacol 2006;108(2):198-203.
CrossRef - Guo L., Ye C., Chen W., Ye H., Zheng R., Li J., Yang H., Yu X and Zhang D. Anti-inflammatory and analgesic potency of carboxyamidotriazole, a tumorostatic agent. J Pharmacol Experimental Therapeutics 2008;325(1):10-16.
CrossRef - Morris CJ. Carrageenan-induced paw edema in the rat and mouse. In: Winyard PG, Willoughby DA, editors. Methods in Molecular Biology: 225: Inflammation Protocols. Totowa: Humana Press Inc; 2003. p.115-121.
CrossRef - Sun Y-G, Lundeberg T and Yu L-C. Involvement of endogenous beta-endorphin in antinociception in the arcuate nucleus of hypothalamus in rats with inflammation. Pain 2003;104(1-2):55-63.
CrossRef - Somchit M.N., Solihah M.H., Israf D.A., Zuraini A., Arifah A.K and Jais A.M.M. Effects of three local malaysian Channa spp. fish on chronic inflammation. Oriental Pharmacy and Exp Med 2004;4(2):91-94.
CrossRef - Pountos I., Georgouli T., Bird H and Giannoudis P.V. Nonsteroidal anti-inflammatory drugs: prostaglandins, indications, and side effects. International Journal of Interferon, Cytokine and Mediator Research 2011;3(1):19-27.
CrossRef - Yong Y.K., Arifah A.K., Sukardi S., Roslida A.H., Somchit M.N and Zuraini A. Bixa orellana leaves extract inhibits bradykinin-induced inflammation through suppression of nitric oxide production. Medical Principles and Practice 2011;20(2):142-146.
CrossRef - Tao F., Tao Y.X., Zhao C., Dore S., Liaw W.J., Raja S.N and Johns RA. Differential roles of neuronal and endothelial nitric oxide synthases during carrageenan-induced inflammatory hyperalgesia. Neuroscience 2004;128(2):421-430.
CrossRef - Li L., Rossoni G., Sparatore A., Lee L.C., Del Soldato P and Moore PK. Anti-inflammatory and gastrointestinal effects of a novel diclofenac derivative. Free Radical Biology and Medicine 2007;42(5):706-719.
CrossRef - Loram LC, Fuller A, Fick LG, Cartmell T, Poole S and Mitchell D. Cytokine profiles during carrageenan-induced inflammatory hyperalgesia in rat muscle and hind paw. The Journal of Pain 2007;8(2):127-136.
CrossRef - Saraiva M and O’Garra A. The regulation of IL-10 production by immune cells. Natural Reviews Immunology 2010;10(3):170-181.
CrossRef - Vane JR and Botting RM. The mechanism of action of aspirin. Thrombosis Research 2003;110(5-6):255-258.
CrossRef - Noguchi M, Kimoto A, Gierse JK, Walker MC, Zweifel BS, Nozaki K and Sasamata M. Enzymologic and pharmacologic profile of loxoprofen sodium and its metabolites. Biological and Pharmaceutical Bulletin 2005;28(11):2075-2079.
CrossRef