Manuscript accepted on :07-11-2023
Published online on: 02-01-2024
Plagiarism Check: Yes
Reviewed by: Dr. Revathi Shenoy
Second Review by: Dr. Husam Al-Hraishawi
Final Approval by: Dr. Patorn Piromchai


Aghaida M. Abusuliman1, Abeer S. Amin 2, Mona A. Higo3 , Amna A. Saddiq1 and Hend M. Tag4,5
1Department of Biology, College of Science and Arts at Khulis, University of Jeddah, Saudi Arabia.
2Department of Botany, Faculty of Science, Suez Canal University, Egypt;
3Department of Zoology, Faculty of Science, Al-Arish University, North Sinai, Egypt;
4College of Applied Medical Sciences, University of Jeddah, Saudi Arabia.
5Department of Zoology, Faculty of Science, Suez Canal University, Egypt.
Corresponding Author E-mail:hend_taha@science.suez.edu.eg
DOI : https://dx.doi.org/10.13005/bpj/2814
Abstract
The Red Sea coastline is known for its diverse range of seaweeds, which have been hypothesized to possess therapeutic attributes. The primary aim of this research was to evaluate the antifungal and anti-inflammatory characteristics of the ethanol crude extract obtained from two types of algae, namely Padina pavonica (P. pavonica) and Laurencia catarinensis (L. catarinensis) from the Red Sea. It was revealed from FTIR analysis of P. pavonica and L. catarinensis ethanolic extracts that the strong band at 3366 cm−1 and 3339 cm−1 corresponds to alcoholic O-H stretching in both extracts. GC-MS phytoconstituent profile of the extract was dominant with a wide range of chemical classes mainly steroids, terpenes, fatty acids, fatty acid esters, retinoid derivatives, alcohols, carotenoids and alkanes. The results of the antifungal activity assay indicate that P. pavonica had the strongest activity against Aspergillus fumigatus, with a mean value of 23.50 ± 0.55 mm. Similarly, L. catarinensis demonstrated significant antifungal activity against Candida albicans, with a mean value of 21.00 ± 2.64 mm. The hemolysis exhibited a positive correlation with the dosage of extracts, with an observed increase in hemolysis as the dosage increased. Besides the antiinflammatory activity of the algal extracts induced by carrageenan demonstrating a dose-dependent relationship between the inhibition of paw edema and the dosage of the extract.
Keywords
Antifungal activities; brown algae; carrageenan; histological analysis; Inflammation; paw edema
Download this article as:
Copy the following to cite this article: Abusuliman M, Amin A. S, Higo M. A, Saddiq A. A, Tag H. M. Phytochemical Composition, Antimycotic and In Vivo Antiinflammatory Activities of Marine Algae Inhabiting South Jeddah Seashore, Red Sea, Saudi Arabia. Biomed Pharmacol J 2023;16(4). |
Copy the following to cite this URL: Abusuliman M, Amin A. S, Higo M. A, Saddiq A. A, Tag H. M. Phytochemical Composition, Antimycotic and In Vivo Antiinflammatory Activities of Marine Algae Inhabiting South Jeddah Seashore, Red Sea, Saudi Arabia. Biomed Pharmacol J 2023;16(4). Available from: https://bit.ly/3S5oUzz |
Introduction
The oceans harbor a wealth of biologically active compounds, with macroalgae and microalgae emerging as particularly abundant producers that hold great potential for their significant medicinal properties 1. In the realm of algae, we primarily encounter two distinct categories: microalgae, which inhabit various marine environments such as benthic zones, coastal areas, and the vast expanses of the open sea, and macroalgae, commonly referred to as seaweeds, which thrive predominantly along coastal regions. There is a rich diversity of macroalgae or seaweed species, exceeding 150,000, that have evolved and inhabit various marine ecosystems across the globe, including both temperate and tropical waters. In the coastal regions of our planet, there is a rich diversity of marine seaweeds, with approximately 8000 species documented thus far. These remarkable organisms can be found thriving at various depths, even reaching depths of up to 270 meters in the vast oceanic expanse 2.
The coastal area of the Red Sea, particularly around the city of Jeddah in the Kingdom of Saudi Arabia, exhibits a remarkable abundance and variety of seaweed species 3. A diverse assemblage of non-angiosperm organisms, known as macroalgae, thrives as a community of photosynthetic, non-flowering entities within the marine environment4. Macroalgae, also known as seaweeds, are fascinating eukaryotic organisms that inhabit both marine and freshwater environments. Due to their potential as a valuable source of critical bioactive chemicals, conservation biologists have recently taken an interest in these amazing species. Macroalgae are distinguished from their terrestrial counterparts by a number of unique features, including the lack of roots, leaves, and vascular systems. 5. Chlorophyta, Phaeophyta, and Rhodophyta are the three major divisions of seaweeds and macroalgae based on pigmentation. Green algae are classified as Chlorophyta, brown algae as Phaeophyta, and red algae as Rhodophyta. Phaephyta are characterized by the presence of the pigment fucoxanthin, whereas Chlorophyta possesses the pigments chlorophyll a and chlorophyll b, as well as lutein, zeaxanthin, violaxanthin, neoxanthin, and β-carotene. On the other hand, Rhodophyta exhibit the pigments phycobiliproteins, lutein, zeaxanthin, and β-carotene 6. To fulfill their role as a source of bioactive substances, seaweeds create a wide range of secondary metabolites. These chemicals have a wide variety of biological effects, including anticancer, antiviral, antifungal, antibacterial, cytotoxic, antidermatophytal, phytotoxic, and antiproliferative activity 7.
Fungal infections 8,9 and inflammation 10 have emerged as significant public health issues, demanding increased attention and research. The prevalence of fungal infections, such as candidiasis and aspergillosis, has been on the rise, affecting individuals of all ages and immune statuses 11. These infections can range from mild to severe, sometimes leading to life-threatening complications. Furthermore, chronic inflammation, often associated with conditions like arthritis and inflammatory bowel disease, can significantly impact an individual’s quality of life. Recognizing the growing impact of these conditions, healthcare authorities and researchers are working diligently to develop effective prevention strategies, diagnostic tools, and innovative treatments to address these public health challenges and improve patient outcomes.
Currently, the treatment choices for inflammatory diseases are limited to anti-inflammatory medications and steroid hormones. Given the numerous risks that have been reported in relation to anti-inflammatory medications that specifically target COX and LOX enzymes, it is imperative to identify alternative targets for the treatment of inflammatory illnesses 12. Individuals afflicted with inflammatory diseases can derive advantages from the inherent and effective safeguard offered by complementary and alternative therapy.Hence, the development of an extensive repository of new chemicals is important in order to effectively address these illnesses. The primary aim of this investigation was to evaluate the chemical composition, antifungal effectiveness, and anti-inflammatory properties of the prevailing marine algae species discovered along the coastline of the Red Sea in Jeddah, Saudi Arabia. The region under consideration is known for its abundance of brown algae and red algae, both of which have been identified as valuable reservoirs of bioactive chemicals that exhibit noteworthy biological properties.
Materials and Methods
Algal materials
The algae utilized in this investigation were. Padina pavonica and Laurencia catarinensis. They were collected by handpicking from south jeddah Seashore, Red Sea, KSA. The research encompasses the coastal region of Jeddah, located along the Red Sea in Saudi Arabia within Latitude 21°14’23.8″N and Longitude 39°08’25.8″E (Figure 1), The collection encompassed a distance of 10 kilometers. The samples were initially subjected to thorough washing with sea water to eliminate epiphytes and other particulate matter. Subsequently, they were promptly transported to the laboratory in plastic bags containing water. Upon arrival, the samples underwent a triple rinsing process with tap water and distilled water to eliminate sand, salt, and other impurities. Subsequently, the specimens were subjected to a desiccation process within a sheltered environment, maintaining ambient temperature conditions. The dried algae were ground into a fine powder using an electric mixer and thereafter kept at a temperature of 4 °C for future testing protocols.
Identification of algal species
The identification of the algae species was conducted using a microscope, whereby their morphological traits were observed and compared to taxonomic references in accordance with the descriptions provided by previous studies 13,14 saved in Biology Department Lab, Faculty of Science, King Jeddah University (Khulis), Saudi Arabia (Figure 2).
![]() |
Figure 1: The study area and sampling site location. |
![]() |
Figure 2: (A) Padina pavonica and (B) Laurencia catarinensis |
Preparation of algal crude extracts.
A total of 100 grams of algal powder from each individual sample underwent the process of extraction utilizing a 75% ethanol solution for a duration of 48 hours. The solvent was subjected to filtration and subsequent concentration at a temperature of 40°C under pressure utilizing a rotary evaporator 15. The yield of sticky residue obtained from the raw material was determined to be 9%. The adhesive residue was stored at a temperature of -20°C in order to facilitate future examination.
Fourier Transmission Infra-Red (FT-IR) spectroscopic
In order to perform an analysis on the FTIR spectrum of algal extract, the ethanol extract was subjected to centrifugation at a rotational speed of 10,000 revolutions per minute (rpm) for a period of 30 minutes. To ensure complete removal of any residual proteins and enzymes, the pellet underwent a triple wash procedure utilizing deionized water. Following that, a small quantity of dehydrated powder was finely ground together with potassium bromide (KBr) to produce a homogeneous mixture. The Fourier Transform Infrared (FTIR) spectrum of the sample was acquired utilizing a Perkin Elmer Spectrum GX Range Spectrometer situated at Bridgeport Avenue, USA. The observed spectral range spanned from 400 to 4000 cm-1.
Phytochemical screening for crude extract using Gas chromatography mass spectrometry (GC–MS):
The algal ethanolic extract was analyzed using the Thermo Polaris Q Ion Trap GC–MS Spectrometer, which is located in Hertfordshire, UK. This analysis method has been previously demonstrated16. The gas chromatograph was connected to a mass spectrometer under the specified experimental parameters: In order to facilitate GC-MS detection, The utilization of electron impact ionization, characterized by an ionization energy of 70 electron volts (eV), is suggested. The experiment utilized helium as the carrier gas, which had a high purity level of 99.99 percent. The flow rate was consistently maintained at 1.21 milliliters per minute. The temperature of the injector was modified to 200°C, while the temperature of the mass transfer line was set to 240°C. The oven’s temperature was calibrated within a range of 70 to 220°C, with a consistent rate of change of 10°C per minute. Following this calibration, the temperature was maintained at a constant level for a period of two minutes. Following this, the temperature was further increased to reach 300°C, and this elevated temperature was sustained for a period of 10 minutes. A volume of 2 microliters (μl) of diluted samples was introduced into the system using manual injection in the splitless mode. A split ratio of 1:40 was utilized, and the mass scan range was configured to span from 50 to 650 atomic mass units (amu). The temporal duration required for the GC-MS Spectrometry procedure typically manifests itself as approximately fifty minutes. The data was analyzed using the Finnigan Xcalibur 2.0 software, which is specifically designed for collecting and processing data within the ThermoQuest LC/MS Division. The relative concentration of different constituents within the tested extracts was determined using the normalised peak area percentage. Utilizing the spectrometer database from esteemed sources such as the Wiley and NIST Libraries, and further supported by the determination of the Kovat index, a valuable tool for converting retention periods into system-independent constants, NIST diligently compiles an extensive Kovat index database.
In vitro Antifungal activity of algal extract:
Fungal source and culture condition
In the study, a collection of three reference pathogenic fungal strains was employed. These strains encompassed both unicellular fungi, specifically Candida albicans ATCC 10231, as well as filamentous fungi, represented by Aspergillus fumigatus ATCC 1022 and Aspergillus Niger ATCC. The test organisms were introduced into a culture medium known as Potato Dextrose broth and the specimen was subjected to incubation within an incubator that was configured at a temperature of 37°C. This was done to allow the organisms to grow and reach the turbidity level is comparable to the 0.5 McFarland standards. A suspension of pathogenic fungi, with a concentration of 1.5 × 108 colony forming units per milliliter (cfu/ml), was evenly distributed onto Potato Dextrose Agar (PDA). Preparations of extracts with a concentration of 10 mg/ml were made using distilled water (DW) as the solvent. Following that, cylindrical openings with a diameter of 6 millimeters were created in the agar medium. These openings were then filled with 100 microliters of the extract being tested. Subsequently, the extract was allowed to spread into the surrounding media at room temperature for a period of thirty minutes. The plates were incubated at a temperature of 37°C for a period of 48 hours. while being maintained in an upright orientation. For the negative controls, wells were treated with an equivalent volume of DW. As for the positive control, a standard antibiotic ketoconazole (25 µg/ml) was used specifically for fungi 17. Following incubation, the diameter of the existing transparent zoon was quantified in millimeters. The triplicates were conducted for every extract in relation to each of the test organisms. The data were reported in the form of an average value accompanied by the standard deviation.
Determination of hemolytic assay
Blood was collected from a healthy female volunteer into laboratory tubes containing 3.2% sodium citrate. The blood specimen separated by centrifugation at 2000 rpm, 4oC for 15 min. after removing the supernatant, the cells were washed 3 times with 5ml normal saline solution (0.9% NaCl) followed with 3min of centrifugation at 6000 rpm and 4oC. 5% blood suspension was prepared by adding 500 μl of precipitate blood cells and 9.5ml normal saline then, shake it gently a few times to get it ready for use. For positive control, 3 mL of distilled water and for negative control, 3 mL of normal saline were added respectively to 500 μL of 5% blood suspension while for Samples, Serial dilutions were performed of each extract using saline solution as solvent that’s resulting in six concentrations (1000, 500, 250, 125, 62.5, 31.25 μg/1mL.), so then 3 mL of normal saline and 500 μL of 5% blood suspension were added to 500 μL of tested extracts solutions, it was prepared for each of six concentrations. The tubes were subjected to gentle agitation and placed in an incubator set at a temperature of 35°C for a duration of 60 minutes. Subsequently, they were subjected to centrifugation at a speed of 2500 revolutions per minute (rpm) and a temperature of 4°C for a period of 10 minutes. The measurement of absorbance at a wavelength of 540 nm was conducted using a UV-Vis spectrophotometer for the supernatants 18. The quantification of hemolysis for each fraction was determined by employing the following mathematical expression:

In vivo anti-inflammatory activity
Animals
The study employed male albino rats weighing between 60 and 65 grams. The organisms were housed within synthetic enclosures constructed of plastic materials, with environmental conditions carefully regulated to approximate a thermal range of approximately 25°C and a relative humidity level ranging from 50% to 60%. These conditions were subjected to a cyclic pattern, alternating every 12 hours. Every individual rat was provided with unrestricted access to a standard diet and water. The rats were transported to the laboratory approximately one hour before the commencement of the experimental trials. Before the dose was administered, the overall health of the rats was evaluated by observing their movements, presence of edema, occurrence of diarrhea, and presence of ulceration. This assessment was conducted over a period of 3 days in a laboratory setting to ensure proper acclimatization. The experimental animals were housed under suitable laboratory circumstances for a duration of two weeks prior to the commencement of the experimental procedure. This was carried out in compliance with the rules set forth by the Animal Care and Use Committee (ACUC) at King Fahad Medical Research Center, specifically protocol No. ACUC -23-03-04.
The study involved a sample of 30 Wistar rats, which were separated into 6 groups of equal size, with each group including 5 individuals (n=5). The negative control group was administered a vehicle consisting of distilled water at a dosage of 10ml per kilogram. The experimental group designated as the positive control received an exclusive injection of carrageenan. The remaining four experimental groups were subjected to the management of tested algal extract at levels of 100 and 200 mg/kg. The delivery of tested extracts administrated orally via oral gavage, one hour prior to the injection of carrageenan. A suspension of carrageenan (0.1 mL of 2% w/v) was prepared using normal saline and subsequently administered beneath the aponeurosis of the planter region of the right hind paw of rats. following the methodology19, This procedure was employed to induce hind paw edema in the experimental rats.
Acute toxicity study of the crude extracts
Acute toxicity investigations of the extract were conducted on rats 20. Two groups of controls and three experimental groups were formed using twenty male albino rats weighing 85–100 g. Five animals were involved in each group. While the control group was given water, the experimental group was given extract via gavage using metallic gastric needle at singular dosage of 100, 1000, or 3000 mg/kg of the animal’s weight. Alterations in skin and fur, eyes, mucous membranes, musculature, and respiratory, symptoms were monitored in all animal groups for the first 4 hours after dose administration on the day of treatment, and once daily on days 2 to 14 consecutive days.
Carrageenan-induced Paw Edema
The anti-inflammatory activity of crude extract using carrageenan-induced paw edema model was performed 19, we compared the protective effects of Padina pavonica and Laurencia catarinensis against acute inflammation. Injecting 0.1 ml of 2% carrageenan into the footpad of each rat’s hind paws an hour after oral administration of different medicines causes acute inflammation. The overall size of the sub-plantar space was measured before and 1, 2, 3, 4, 5, 6, and 24 hours after the injection of carrageenan to induce oedema. The lateral and anteroposterior diameters of the hind paw were measured with a caliper. The geometric formula used to calculate the ankle circumference was as follows: circumference = 2 (sqrt (a2 + b2/2)), where a and b are the latero-lateral and anteroposterior axes, respectively. Extracts were measured for their ability to suppress activity compared to a 100% positive control. The formula for determining the edema-inhibition percentage (%) is as follows:
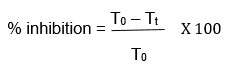
Where Tt is the thickness of paw of rats given test extract at a particular time and To is the paw thickness of rats of control group at the same time.
Histopathological Examination
The animals were humanely euthanized using an excessive amount of anesthetics, following ethical guidelines. Subsequently, the feet affected by carrageenan-induced edema, specifically the “right-hind paws,” were carefully excised and immersed in a 10% formalin solution for fixation. The specimen underwent paraffin embedding, followed by sectioning, staining with hematoxylin eosin, and subsequent examination using a light microscope 21,22. The histological analysis was conducted by evaluating the presence of edema and inflammatory cell infiltrate in both epithelial and connective tissues.
Results
Fourier Transmission Infra-Red spectroscopic
The FTIR spectra of Padina pavonica ethanolic extract is shown in Table 1, Figure 3. The spectra showed bands at 3366, 2923, 2855, 1573, 1417 and 1040 cm−1. The strong band at 3366 cm−1corresponds to alcoholic O-H stretching. The weak band at 2923 and 2855 cm−1 are owing to the C-H stretching. The sharp band at 1573 cm−1 supposed to be N-H bending of amine group. The band 1417 owing S=O stretching. The lower frequency bands at 1040 cm−1 can be assigned to CO-O-CO stretching (anhydride). The FTIR spectra of Laurencia catarinensis extract is shown in Table 1, Figure 4. The spectra showed bands at 3339, 2919, 1635, 1413 and 1034 cm−1. The strong band at 3339 cm−1corresponds to alcoholic O-H stretching (intermolecular bonded). The band at 2919 cm−1 is owing to the N-H stretching. The medium band at 1635 cm−1 supposed to be C=C stretching (alkene). The band 1413 and 1034 are owing S=O stretching.
Phytochemical characterization using gas chromatography-mass spectrometry (GC–MS).
The identification of phytochemicals in the extracts of Padina pavonica and Laurencia catarinensis was accomplished through a comparative analysis of their mass spectra with those of reference compounds archived in the GC-MS library (Figure 5 and 6). A total of 34 phytochemical compounds were detected in the ethanolic extract of Padina pavonica, whereas the ethanolic extract of Laurencia catarinensis included 25 recognized compounds. The identification and quantification of phytochemical compounds are influenced by multiple criteria, such as the measurement of peak area, retention duration, molecular weight, and molecular formula. Gas chromatography-mass spectrometry is a very effective analytical methodology that synergistically integrates the separation capabilities of gas chromatography with the detection and identification capabilities of mass spectrometry. The study of the extract obtained from Padina pavonica revealed that the primary chemical present was Stigmasterol, accounting for 18.38% of the total composition. This was followed by 3-O-Acetyl-6-methoxy-cycloartenol at 14.61%, Fenretinide at 7.79%, Hexadecanoic acid, ethyl ester at 7.77%, and 9-Octadecenoic acid ethyl ester at 5.59% and Dotriacontane (2.15%). Remaining constituent chemical compounds were less than five percentages as summarized in Table 2. While that of Laurencia catarinensis extract displayed that the major compound was Stigmasta-5,24(28)-dien-3-ol,(3á,24Z)- (12.51 %) followed by n-Hexadecanoic acid (9.29%), 1-Heptatriacotanol (7.42%), cholestanoid (5.77%), 9,12-Octadecadienoyl chloride,(Z,Z)- (2.87%), Rhodopin (2.77%). Remaining constituent chemical compounds were less than 2 percentages as summarized in Table 3.
Anti-fungal activity of algal extracts
Secondary metabolites of Padina pavonica and Laurencia catarinensis extracts were tested for antifungal activities against human pathogenic microbes; unicellular fungi; Candida albicans ATCC 10231, filamentous fungi; Aspergillus fumigatus ATCC 1022 and Aspergillus Niger (RCMB 002005) were determined by agar well diffusion assay. The antifungal effect of ethanolic extracts of Padina pavonica and Laurencia catarinensis were quantified by zone of inhibition. The extracts of tested algae at 100µg/mL demonstrated varying degrees of antifungal activity against human harmful microorganisms. One-way analysis of variance was done to determine if zone of inhibition means of different tested extracts were significantly different from each other against human pathogenic microbes. The analysis indicated that there was significant differences recorded in zone of inhibition between the different tested extracts.
The extract of Padina pavonica revealed significant antifungal activity against Candida albicans (22.00 ± 4.58 mm), Aspergillus fumigatus (23.50 ± 0.55 mm), Aspergillus Niger (18.43 ± 1.69 mm) as compared with control (Ketoconazole) of 22.56±2.89, 18.16±1.70 and 17.24±2.80 respectively. While that of Laurencia catarinensis against Candida albicans is 21.00±2.64, Aspergillus fumigatus is 12.00±1.00 and Aspergillus Niger is 12.00±1.00 as compared with control (Ketoconazole) of 22.56±2.89, 18.16±1.70 and 17.24±2.80 respectively. The antifungal activity showed that the highest activity values were 23.50 ± 0.55 mm against Aspergillus fumigatus for Padina pavonica and 21.00 ± 2.64 mm against Candida albicans for Laurencia catarinensis (Table 4).
Hemolytic Activity of algal extracts
Haemolytic activity of the Padina pavonica and Laurencia catarinensis extracts are expressed in percentage hemolysis and investigated as mean ± standard deviation of three replicates. All the concentration exhibited hemolytic effect toward human Erythrocytes as compared with positive control (P<0.05) (Figure 7 and Figure 8). Cytotoxicity against erythrocytes, was tested at concentrations extending from 1000 to 31.25 µg/ml, as shows the hemolysis is increasing as the dose of extracts increased whereas, at a concentration 31.25 µg/ml the hemolysis was 12.76% for Padina pavonica while for Laurencia catarinensis was 3.085%, while at 1000 g/ml, Padina pavonica extract possess maximum hemolytic activity (63.4%) and that of Laurencia catarinensis extracts possess (46.7%) at the same concentration. Thus, Laurencia catarinensis extractexhibited lower toxicity as compared with Padina pavonica.
Table 1: Initial assignment of bands found in Fourier Transform Infrared spectra of Padina pavonica and Laurencia catarinensis extracts.
Main peak (cm-1) (Peaks for Padina pavonica in brackets) |
Typical band assignment from the literature |
Wave number range (cm-1) |
3327 (3366.04) |
Water v(O-H) stretching Protein v(N-H) stretching (amide A) |
3029-3639 |
2928 (2923.14) |
Lipid – carbohydrate Mainly vas(CH2) and vs(CH2) stretching |
2809-3012
|
2928 (2855.16) |
||
1543 (1573.84) |
Protein amide II band mainly δ(N-H) bending and v(C-N) stretching |
1481-1585 |
1389 (1417.28) |
Protein δs(CH2) and δs(CH3) bending of methyl Carboxylic Acid vs(C-O) of COO groups of carboxylates Lipid δs(N(CH3)3) bending of methyl |
1357-1423 |
1036 (1040.30) |
Carbohydrate v(C-O-C) of polysaccharides |
980-1072 |
Main peak (cm-1) (Peaks for Laurencia catarinensis in brackets) |
Typical band assignment from the literature |
Wave number range (cm-1) |
3327 (3339.26) |
Water v(O-H) stretching Protein v(N-H) stretching (amide A) |
3029-3639 |
2928 (2919.02) |
Lipid – carbohydrate Mainly vas(CH2) and vs(CH2) stretching |
2809-3012
|
1649 (1635.64) |
Protein amide I band Mainly v(C=O) stretching |
1583-1709 |
1389 (1413.16) |
Protein δs(CH2) and δs(CH3) bending of methyl Carboxylic Acid vs(C-O) of COO groups of carboxylates Lipid δs(N(CH3)3) bending of methyl |
1357-1423 |
1036 (1034.12) |
Carbohydrate v(C-O-C) of polysaccharides |
980-1072 |
![]() |
Figure 3: FTIR spectroscopy within the wavelength range of 400–4000 cm−1 to |
![]() |
Figure 4: FTIR spectroscopy within the wavelength range of 400–4000 cm−1 |
Table 2: Identification of phytocomponents of ethanolic extract Padina pavonica using GC-MS analysis.
NO. |
Compound name |
Retention time |
Molecular formula |
Molecular weight |
Percent abundant |
1. |
Glycidyl oleate |
23.16 |
C21H38O3 |
338 |
0.41 |
2. |
6,9,12,15-Docosatetraenoic acid,Methyl ester |
25.37 |
C23H38O2 |
346 |
0.22 |
3. |
Cis-11-Eicosenoic acid |
25.44 |
C20H38O2 |
310 |
0.22 |
4. |
Hexadecanoic acid, methyl ester |
26.3 |
C17H34O2 |
270 |
1.65 |
5. |
Hexadecanoic acid, ethyl ester |
27.63 |
C18H36O2 |
284 |
7.77 |
6. |
methyl 6,9,12-octadecatrienoate |
28.35 |
C19H32O2 |
292 |
0.29 |
7. |
10-Octadecenoic acid, methyl ester |
29.58 |
C19H36O2 |
296 |
1.17 |
8. |
Phytol |
29.78 |
C20H40O |
296 |
1.33 |
9. |
Oxiraneundecanoic acid, 3-pentyl-,Methyl ester, cis- |
30.09 |
C19H36O3 |
312 |
0.17 |
10. |
9,12,15-Octadecatrienoic acid,2,3-dihydroxypropyl ester, (Z,Z,Z)- |
30.42 |
C21H36O4 |
352 |
1.73 |
11. |
Butyl 9,12-octadecadienoate |
30.65 |
C22H40O2 |
336 |
0.64 |
12. |
9-Octadecenoic acid ethyl ester |
30.79 |
C20H38O2 |
310 |
5.59 |
13. |
Octadecanoic acid, ethyl Ester |
31.3 |
C20H40O2 |
312 |
0.45 |
14. |
9,12,15-Octadecatrienoic acid,2,3-dihydroxypropyl ester, (Z,Z,Z)- |
32.29 |
C21H36O4 |
352 |
0.57 |
15. |
Z-(13,14-Epoxy)tetradec-11-en-1-olAcetate |
32.63 |
C16H28O3 |
268 |
0.19 |
16. |
Arachidonic_acid |
33.36 |
C22H36O2 |
332 |
1.53 |
17. |
Butyl 5,8,11,14,17-eicosapentaenoate |
33.46 |
C24H38O2 |
358 |
0.34 |
18. |
9,12,15-Octadecatrienoic acid,2,3-dihydroxypropyl ester, (Z,Z,Z)- |
33.74 |
C21H36O4 |
352 |
0.18 |
19. |
E,E,Z-1,3,12-Nonadecatriene-5,14-dIol |
34.25 |
C19H34O2 |
294 |
0.35 |
20. |
5-hydroxy-7-methoxyflavanone |
34.66 |
C16H14O4 |
270 |
1.15 |
21. |
Hexadecanoic acid,1-(hydroxymethyl)-1,2-ethanediyl Ester |
36.57 |
C35H68O5 |
568 |
0.45 |
22. |
Bis(2-ethylhexyl)Phthalate |
36.77 |
C24H38O4 |
390 |
4.81 |
23. |
(E)-1-(2-Hydroxy-4,6-dimethoxyphe Nyl)-3-phenylprop-2-en-1-one |
38.22 |
C17H16O4 |
284 |
0.76 |
24. |
Isochiapin b |
39.09 |
C19H22O6 |
346 |
3.27 |
25. |
9-octadecenoic acid |
39.38 |
C18H34O2 |
282 |
0.63 |
26. |
Fenretinide |
40.43 |
C26H33NO2 |
391 |
7.79 |
27. |
7,8-Epoxylanostan-11-ol, 3-acetoxy- |
41.1 |
C32H54O4 |
502 |
0.23 |
28. |
Ethyl iso-allocholate |
41.88 |
C26H44O5 |
436 |
2.18 |
29. |
Stigmasterol |
42.49 |
C29H48O |
412 |
18.38 |
30. |
1-heptatriacotanol |
43.04 |
C37H76O |
536 |
1.88 |
31. |
Dotriacontane |
43.31 |
C32H66 |
450 |
5.12 |
32. |
Ç-Sitosterol |
43.83 |
C29H50O |
414 |
2.4 |
33. |
3-O-Acetyl-6-methoxy-cycloartenol |
44.12 |
C33H54O3 |
498 |
14.61 |
34. |
Rhodopin |
45.37 |
C40H58O |
554 |
1.02 |
Table 3: Identification of phytocomponents of ethanolic extract Laurencia catarinensis using GC-MS analysis.
NO. |
Compound name |
Retention time |
Molecular formula |
Molecular weight |
Percent abundant |
1 |
1,25-Dihydroxyvitamin D3, |
23.15 |
C30H52O3Si |
488 |
0.61 |
2 |
13-Heptadecyn-1-ol |
24.5 |
C17H32O |
252 |
0.61 |
3 |
1-Heptatriacotanol |
41.54 |
C37H76O |
536 |
7.42 |
4 |
5,8,11,14-Eicosatetraenoic acid, methyl ester, (all-Z)- |
33.35 |
C21H34O2 |
318 |
0.83 |
5 |
7-Methyl-Z-tetradecen-1-ol acetate |
25.37 |
C17H32O2 |
268 |
0.66 |
6 |
9,12-Octadecadienoyl chloride,(Z,Z)- |
30.41 |
C18H31ClO |
298 |
2.87 |
7 |
9-Hexadecenoic acid |
26.76 |
C16H30O2 |
254 |
0.61 |
8 |
9-Octadecenoic acid,1,2,3-propanetriyl ester, (E,E,E)- |
32.24 |
C57H104O6 |
884 |
0.41 |
9 |
Androstan-17-one |
33.45 |
C21H34O2 |
318 |
0.3 |
10 |
Cholestanoid |
43.81 |
C27H46O |
386 |
5.77 |
11 |
Cycloheptasiloxane, tetradecamethyl- |
16.31 |
C14H42O7Si7 |
518 |
0.13 |
12 |
Espatulenol |
18.84 |
C15H24O |
220 |
0.17 |
13 |
Ethyl Oleate |
30.77 |
C20H38O2 |
310 |
1.63 |
14 |
Hexadecanoic acid, ethyl ester |
27.62 |
C18H36O2 |
284 |
1.66 |
15 |
Linoleic acid ethyl ester |
34.66 |
C20H36O2 |
308 |
1.09 |
16 |
methyl 13-methylpentadecanoate |
26.3 |
C17H34O2 |
270 |
0.61 |
17 |
n-Hexadecanoic acid |
27.15 |
C16H32O2 |
256 |
9.29 |
18 |
Oleic Acid |
29.57 |
C18H34O2 |
282 |
0.68 |
19 |
Oxiraneoctanoic acid, |
30.09 |
C18H34O3 |
756 |
0.15 |
20 |
Phytol |
29.78 |
C20H40O |
296 |
1.83 |
21 |
Retinoic acid, methyl ester |
37 |
C21H30O2 |
314 |
0.75 |
22 |
Rhodopin |
43.56 |
C40H58O |
554 |
2.77 |
23 |
Stigmasta-5,24(28)-dien-3-ol,(3á,24Z)- |
36.3 |
C29H48O |
412 |
4.05 |
24 |
trans-13-Octadecenoic acid |
30.89 |
C18H34O2 |
282 |
0.28 |
25 |
Dammarenediol-II |
39.41 |
C30H52O2 |
444 |
2.72 |
![]() |
Figure 5: A chromatogram of an ethanolic sample of Padina pavonica was made using GC-MS spectrometry, the main chemicals can be seen in the GC-MS spectrum at retention time 0.00–45 min of. |
![]() |
Figure 6: A chromatogram of an ethanolic sample of Laurencia catarinensis was made using GC-MS spectrometry, the main chemicals can be seen in the GC-MS spectrum at retention time 0.00–45 min of. |
Table 4: Zone of Inhibition (mm) for Padina pavonica and Laurencia catarinensis against various pathogenic. microorganisms
Treatments (100 µg/ml) |
Candida albicans |
Aspergillus fumigatus |
Aspergillus Niger |
Padina pavonica extract |
22.00 ± 4.58a |
23.50 ± 0.55 a |
18.43 ± 1.69 a |
Laurencia catarinensis extract |
21.00 ± 2.64 a |
12.00 ± 1.00 b |
12.00 ± 1.00 b |
Positive control (Ketoconazole) |
22.56 ± 2.89 a |
18.16 ± 1.70 c |
17.24 ± 2.80 a |
Data represents as Mean±SD. Different letters are used to denote substantial variations between the treatments. Duncan test was utilized to conduct multiple comparisons of means at a significance level of 0.05.
Anti-inflammatory Activity
Invivo Acute toxicity study of the crude extracts
Padina pavonica and Laurencia catarinensis extracts was investigated for in vivo toxicity on experimental rats; injections up to 5000 mg/kg didn’t cause lethal effects. There was no mortality in rats of the tested extract. The behavior and body weight did not change in the control or all treated groups.
Anti-Inflammatory Activity of extracts in carrageenan injected rats
Alteration in paw thickness showed in figure 9 illustrate that Padina pavonica extract significantly reduced paw volume in a dose-dependent manner. Both doses (50 and 100 mg/kg) significantly suppressed paw edema over the course of 24 hours. The most significant reduction in paw edema was observed in the group given 100 mg/kg. In comparison to the Padina pavonica extract, the anti-inflammatory effects of the Laurencia catarinensis extract were significantly reduced.
A study conducted on rats shown that the inhibition of carrageenan-induced paw edema exhibited a dose-dependent relationship over a 24-hour duration subsequent to the intraperitoneal injection of Padina pavonica extract. The reported effects were observed at two different doses, namely 50 mg/kg and 100 mg/kg. The observed percent of inhibition at various time points after treatment with a low dose was found to be 11.52 ± 0.089%, 11.731 ± 0.088%, 12.06 ± 0.62%, 11.171 ± 0.06%, 11.360 ± 0.133%, 11.037 ± 0.113%, and 10.962 ± 0.094% after 1, 2, 3, 4, 5, 6, and 24 hours, respectively. On the other hand, the high dose (100 mg/kg) resulted in inhibition percentages of 11.48 ± 0.066%, 11.55 ± 0.043%, 12.17 ± 0.145%, 10.917 ± 0.094%, 11.111 ± 0.178%, 10.977 ± 0.155%, and 10.749 ± 0.160% after 1, 2, 3, 4, 5, 6, and 24 hours, respectively (Figure 10).
![]() |
Figure 7: Hemolytic activity: Hemolysis (%) of human red blood cells (RBCs) treated with Padina pavonica. Data represented as mean ±SE |
![]() |
Figure 8: Hemolytic activity: Hemolysis (%) of human red blood cells (RBCs) treated with Laurencia catarinensis extract. Data represented as mean ±SE |
Histopathology Analysis
Paw edema after sub-plantar injection of carrageenan in rats was studied histopathologically using HE staining to determine the effects of Padina pavonica and Laurencia catarinensis extracts. Figure 11A shows that the control group showed no signs of cellular infiltration or edema. In contrast, blistering of the epithelial and conjunctive tissues and a large infiltration of inflammatory cells, primarily lymphocytes, were hallmarks of the acute inflammatory response caused by carrageenan in the rat paw (Figure 11B). Treatment with P. pavonica at 50 and 100 mg/kg resulted in a marked decrease in edema and lymphocyte infiltration (Figures 11C and 11D). However, the L. catarinensis -treated group (50 mg/kg) showed no improvements in edema and lymphocytes infiltration (Figure 11E). When L. catarinensis was given to rats with carrageenan-induced edema, a high dose reduced cellular infiltration but had no effect on swelling or congestion (Figure 11F).
Discussion
Seaweeds possess significant potential as a rich reservoir of bioactive chemicals that exhibit pharmacological activities. Consequently, there is a compelling need for comprehensive investigation into their prospective application in the management and avoidance of chronic ailments. Seagrass consists of a diverse range of algae species that have been demonstrated to possess biologically active compounds, including polyphenols, flavonoids, and tannins 23.
A graphical representation of the vibrational and rotational energy levels of molecules in an infrared spectrum is called a Fourier-transform infrared spectroscopy (FTIR) plot. The identity, concentration, and bonding of compounds are only a few examples of the physical and chemical characteristics of substances that can be characterized using FTIR plots. By gathering infrared data from a sample material and graphing it against wavenumber, the plot is made. The molecular makeup and structure of the sample material can then be deduced from the plot that results. FTIR plots offer a thorough examination of the vibrational energy levels of molecules, making them particularly helpful for identifying unidentified substances 24.
The topic of interest pertains to the allocation of band assignments and the dynamics of relationships within the band. The organisms Padina pavonica and Laurencia catarinensis exhibited distinct bands in their FTIR spectra. The process of identification involves comparing the bands observed in the recorded FTIR spectra with those documented in reference material. The employment of work enhanced the functional constituents inherent in the algal extract. In this investigation, the average locations of protein (amides I and II), lipid, and carbohydrate absorption bands were found to align with those reported in the literature for both algae. The FTIR transmittance analysis of the Padina pavonica and Laurencia catarinensis algae species indicates the existence of many chemical groups, including O-H, C-H, N-H, S=O, and CO-O-CO.
![]() |
Figure 9: Alteration in paw thickness (mm) at t = 0,1,2,3, 4,5, and 24 hours. n = 5 (significant at P < 0.05). |
![]() |
Figure 10: Percentage inhibition (%) of paw edema in time-dependent doses of P. pavonica and L. catarinensis extracts. |
![]() |
Figure 11. Histological microphotography of rat paw (A) Saline control group. (B) Carrageenan model group. (C) Group treated with 50 mg/kg of P. pavonica |
The presence of asymmetric C-H stretching vibration is responsible for the faint band observed at 2923 and 2855 cm−1. The presence of aliphatic groups is associated with a notable augmentation in the quantity of hydrocarbons with mid- and long-chain lengths. The presence of discernible lipid signatures within the biomass of Padina pavonica is evident based on the analysis of its FTIR spectrum. The spectra exhibit large and intense bands around 3366 cm−1, which are likely attributed to the stretching vibration of O-H water molecules. The principal amines exhibit a N–H bending vibration at a wavenumber of 1573 cm−1. It is worth noting that these bands are characterized by their higher intensity and narrower linewidth compared to the O–H stretching vibrations of alcohols, which also manifest in the same spectral area. Bands at approximately 1040.30 and 1034.12 cm-1 were observed in certain spectra of algae as well. The observed band locations correspond to the (C-O-C) stretching vibrations associated with polysaccharides.
Regarding phytochemical characterization using GC–MS of tested extracts. In the present study Padina pavonica and Laurencia catarinensis species of brown algae collected from the coast of the Red Sea in Jeddah to analyze its bioactive components. GC-MS analysis of both extracts indicated the existence of 34 phytochemical compounds in the ethanolic extract of Padina pavonica while 25 were identified in the ethanolic extract of Laurencia catarinensis. The identification of phytochemicals was accomplished through the comparison of their mass spectra with those of reference compounds that were archived in the GC-MS library.
The GC-MS analysis of Padina pavonica extract displayed that the major compound was Stigmasterol (18.38%), followed by 3-O-Acetyl-6-methoxy-cycloartenol (14.61%), Fenretinide (7.79%), Hexadecanoic acid, ethyl ester (7.77%), 9-Octadecenoic acid ethyl ester (5.59%) and Dotriacontane (2.15%). While The GC-MS analysis of Laurencia catarinensis extract displayed that the major compound was Stigmasta-5,24(28)-dien-3-ol,(3á,24Z)- (12.51 %) followed by n-Hexadecanoic acid (9.29%), 1-Heptatriacotanol (7.42%), cholestanoid (5.77%), 9,12-Octadecadienoyl chloride,(Z,Z)- (2.87%), Rhodopin (2.77%).
Seaweeds possess a significant abundance of bioactive chemicals that exhibit a favorable safety profile and lack the toxic properties commonly associated with chemical manufactured medications. The components that were found encompassed a diverse array of chemical classes, predominantly consisting of steroids, terpenes, fatty acids, fatty acid esters, retinoid derivatives, alcohols, carotenoids, and alkanes. 25,26.
The substances that have been discovered display a wide range of biological characteristics. It was discovered that the extract made from the captured algae specimens included a considerable amount of stigmasterol. Animal and plant organisms both contain sterols. It is an unsaturated plant sterol used to make semi-synthetic progesterone as a precursor 27. This hormone is highly valuable to human physiology as it plays a crucial role in regulating and facilitating tissue rebuilding mechanisms associated with estrogen effects. In the field of pharmacology, phytosterols like stigmasterol and sitosterol are commonplace. Fucosterol and its derivatives are primarily found in brown seaweeds. Studies have shown that brown seaweeds contain significant amounts of sterols including fucosterol and desmosterol. Extensive documentation indicates that these particular sterols exhibit a propensity for reducing cholesterol levels and diminishing the presence of free triglycerides within the hepatic organ 28.
GC-MS analysis of extracts of Padina pavonica and Laurencia catarinensis, both of which have been credited in the past literature with antioxidant, antibacterial, and anticancer properties, revealed that Stigmasterol, Stigmasta-5,24(28)-dien-3-ol,(3á,24Z)- were the main components of both plants.
Padina pavonica extract had a triterpene with a distinctive high peak in the mass spectral data, and this compound was found to be 3-O-acetyl-6-methoxy-cycloartenol. Brown algae have been found to contain terpenes as well. Halogenated monoterpenes are very useful in a variety of biological processes. The anti-proliferative effect of isolated halogenated monoterpenes was significant. Diterpene phytol has potential as a diuretic, anti-inflammatory, anti-cancer, and anti-microbial agent. Phytol has been shown to have beneficial curative and preventative effects on arthritis 29-31. The findings indicate that chemicals that promote reactive oxygen species, such as phytol, hold potential as a new class of medications for the management of rheumatoid arthritis and maybe other chronic inflammatory conditions.
Studies have documented that the brown algae species Padina pavonica and Laurencia catarinensis exhibit a notable abundance of terpenes and sterols. The results of this study align with other research indicating that several terpenes produced by L. obtusaecies possess noteworthy pharmacological properties, including antiviral, antibacterial , anti-inflammatory and anti-cancer actions 32.
The presence of bioactive chemicals within the fatty acid compounds was detected in both algal extracts, these compounds are n- hexadecanoic acid; hexadecanoic acid, ethyl ester; 9-Octadecenoic acid ethyl ester and 9,12-Octadecadienoyl chloride, (Z, Z). n-Hexadecanoic acid (Palmitic acid), 9-Octadecenoic acid ethyl ester and Hexadecanoic acid ethyl ester, have the property of antioxidant. 9,12-Octadecadienoyl chloride,(Z,Z)- (Lineoleoyl chloride) have been identified from Laurencia catarinensis extract 33. Linolenic acid found in red algae Champia parvula 34.
The ethanolic extracts of brown algae Padina pavonica showed the presence of other compounds such as dotriacontane, belongs to the category of organic compounds referred as alkanes. it has several activities like antimicrobial 35.
To assess the antifungal activity of algal extracts, agar well diffusion method was performed. The clear or inhibition zone developed around well was measured and the antifungal activity is represented by inhibition zone diameter (IZD). In the current study the antifungal activity of P. pavonica and L. catarinensis extracts against some important human pathogens. The current results revealed that, P. pavonica showed potent antifungal activity and inhibited tested fungal species 36. Our results were consistent with earlier study that declared that Padina pavonica showed significant antimicrobial activity 37. The present findings indicate that the L. catarinensis extract exhibits lower values of inhibition zone, measuring 12 mm. Previous research has been conducted to investigate the antibacterial properties of secondary metabolites derived from various species of algae. These studies have demonstrated that the effectiveness of these activities is contingent upon the specific characteristics and composition of the secondary metabolites found within the algae 38.
Padina pavonica and Laurencia catarinensis are two marine algae that have been investigated for their potential antifungal activity. Both species have shown promising results, but the studies indicate that Padina pavonica extract exhibits stronger antifungal activity compared to Laurencia catarinensis extract. Padina pavonica, also referred to as “Peacock’s Tail,” which is a brown algacollected from red sea. Previous study have demonstrated the antimicrobial potential of Padina sp. extract against various microbial species 39. The extracts of tested algae have been shown to inhibit the growth of pathogenic fungi, including Candida species, dermatophytes, and Aspergillus species. The antimicrobial characteristics of Padina pavonica extract are ascribed to its bioactive components, including polyphenols and polysaccharides, which are responsible for its antifungal activity. These substances possess the ability to disrupt the integrity of fungal cell membranes, impede the functioning of fungal enzymes, and interfere with the process of fungal cell reproduction. Consequently, these mechanisms culminate in the inhibition of fungal growth 40.
On the other hand, Laurencia catarinensis is a red alga found Red Seashore, Kingdom of Saudi Arabia. Although Laurencia catarinensis has also shown antifungal activity, the potency of its extract appears to be lower compared to Padina pavonica. Some studies have reported moderate antimicrobial effects of Laurencia sp. extract. However, the exact mechanisms responsible for its antifungal activity are not well understood.
Erythrocytes, the body’s most abundant cell type, have their own unique physical and biochemical properties when it comes to replicating. Because of their role as redox-active oxygen transport mechanisms, erythrocytes are a primary target for hemoglobins and polyunsaturated fatty acids (PUFA). Therefore, oxidation causes hemolysis by damaging lipids and proteins in erythrocyte membranes. Several variables contribute to this mutilation, including radiation, a high concentration of transition metals, oxidative medications, hemoglobinopathies, and erythrocyte antioxidant coordination deficits. When erythrocytes are subjected to toxins like hydrogen peroxide, hemolysis increases 41.
In the present study the ability of Padina pavonica and Laurencia catarinensis extracts against normal human erythrocytes was assessed in order to examine their potential to cause damage to mammalian cells. Extracts exhibited low hemolytic effect on human red blood cells (RBC). Hemolytic percentage was found to be increasing with increase in concentration. Hemolytic activity of crude extracts is expressed in % hemolysis. ethanolic extract of Padina pavonica (at dose 1000 g/ml) possess maximum hemolytic activity (63.4%) while that of Laurencia catarinensis extracts possess (46.7%) at the same concentration. At a concentration 31.25 μg/ml the hemolysis was 12.756% for Padina pavonica while for Laurencia catarinensis was 3.085%.
Green, brown, and red algae have all been found to have bioactive chemicals, such as secondary metabolites having cytostatic, antiviral, anthelmintic, antifungal, and antibacterial activity 42. The algal extracts possess anti-hemolytic activity on human erythrocytes, these activities of both extracts are related to their chemical composition, it may be because of presence of high concentration of terpenes 43.
The agar well diffusion method was employed to evaluate the antifungal activity of algal extracts. The measurement of the clear or inhibition zone surrounding the well was conducted, and the antifungal activity is quantified by the diameter of the inhibition zone (IZD). In the current study the antifungal activity of P. pavonica and L. catarinensis extracts against some important human pathogens. The current results revealed that, P. pavonica showed potent antifungal activity and inhibited tested fungal species. Our results were consistent with earlier study that declared that P. pavonica showed significant antimicrobial activity 39. The current results show that the lower values of inhibition zone belong L. catarinensis extract (12 mm). Extensive research has been conducted on the antibacterial properties of secondary metabolites derived from various algae species. These studies have demonstrated that the effectiveness of these compounds is contingent upon the specific characteristics of the secondary metabolites found within the algae44.
The utilization of the carrageenan-induced rat paw edema model has been extensively employed in the assessment of anti-inflammatory drugs, particularly to evaluate the drug’s antiedematous effect. Carrageenan, a potent chemical compound, is employed to induce the secretion of several inflammatory and proinflammatory agents, such as prostaglandins, leukotrienes, histamine, bradykinin, TNF-α, and similar molecules 45.
The manifestation of acute inflammation exhibits a biphasic trajectory. The early phase is initiated by the liberation of histamine, serotonin, and kinins following the introduction of an inflammatory agent during the initial hours. 46. The second phase is marked by the subsequent liberation of prostaglandin-like molecules within a time period of 2-3 hours. The subsequent phase exhibits reactivity towards both steroidal and nonsteroidal anti-inflammatory agents, which possess clinical utility. Prostaglandins play a pivotal role as the primary causative agents responsible for the initiation and progression of acute inflammation 47.
Both tested agents P. pavonica and L. catarinensis revealed anti-inflammatory activity trough reduction of rat paw edema caused by carrageenan. But P. pavonica revealedpotent activity. The current work aims to investigate the histological aspects of the anti-inflammatory effects in carrageenan-induced rat paw edema. The assessment of acute oral toxicity was also conducted within the scope of this investigation. According to the results of our investigation, it has been determined that the LD50 value of P. pavonica and L. catarinensis exceeds 5000 mg/kg. Based on our findings, the chemical in question can be categorized as a compound with a favorable safety profile and minimal toxicity. Our data indicates that P. pavonica exhibits inhibitory effects on inflammation in the paw tissues of rats, with the extent of inhibition being dependent on the dosage administered. Furthermore, our investigations indicate that the intragastric administration of L. catarinensis demonstrates a comparatively lower degree of anti-inflammatory activity when compared to P. pavonica, when administered at an equivalent dosage level.
P. pavonica may potentially possess an anti-inflammatory compound that exhibits inhibitory effects on prostaglandins and the inflammatory pathway. Previously, certain researchers have also posited the potential anti-inflammatory attributes associated with brown algae. The brown algae Turbinaria decurrens exhibits anti-inflammatory properties, as per scientific observations. 48, which can be attributed to its ability to regulate the levels of enzymatic antioxidants, the master regulator NF-κB, and pro-inflammatory cytokines 49. Furthermore, it has been elucidated that the brown algae Padina tetrastromatica demonstrates anti-inflammatory characteristics through the inhibition of inflammatory response markers’ expression, such as cytokines IL-6, TNF-α, MCP1, and IL1ß. 50.
Another endeavor to elucidate; notwithstanding, The cyclooxygenase and lipoxygenase pathways play a pivotal role in the inflammatory cascade. It has been observed that suppressing cyclooxygenase is more effective in inhibiting carrageenan-induced inflammation compared to inhibiting lipoxygenase. P. pavonica may have exhibited inhibitory effects on the cyclooxygenase enzyme responsible for the synthesis of prostaglandins 51
Conclusion and Recommendation
While there exist conventional therapeutic interventions for various inflammatory conditions, it is postulated that the efficacy of current anti-inflammatory medications may be limited due to their associated adverse effects and relatively modest strength. Thus, alternative therapy research is necessary and required. This study examines the phytochemical content of P. pavonica and L. catarinensis algae extracts. These findings imply that biologically active chemicals that inhibit pathogenic fungus strains like Candida albicans, Aspergillus fumigatus, and Aspergillus Niger may be extracted. These findings support the traditional use of these algae and suggest a potential function in microbial control. P. pavonica and L. catarinensis algae extracts show anti-inflammatory effects and are safe, making them promising carrageenan-induced inflammation treatments.so these seaweeds can be used to develop pathogen-fighting drugs. In conclusion, P. pavonica and L. catarinensis algae extracts may be bioactive chemical reservoirs. These snippets show promise in natural antioxidants and could lead to chronic disease treatments. However, additional fractionation and careful pharmacological assessment are needed to fully investigate the preclinical properties of these extracts and enable their therapeutic use in chronic disease prevention and treatment.
Acknowledgement
None
Conflict of Interest
The authors declare no competing interests.
Funding Sources
This research received no grant from any funding agency.
References
- Kumar A, Naraian R. Chapter 16 – Producers of Bioactive Compounds. In: Gupta VK, Pandey A, eds. New and Future Developments in Microbial Biotechnology and Bioengineering. Amsterdam: Elsevier, 2019:205-221.
- Schroeder ID, Santora JA, Mantua N, Field JC, Wells BK, Hazen EL, Jacox M, Bograd SJ. Habitat compression indices for monitoring ocean conditions and ecosystem impacts within coastal upwelling systems. Ecological Indicators 2022;144:109520.
- Al-Saif SSA-l, Abdel-Raouf N, El-Wazanani HA, Aref IA. Antibacterial substances from marine algae isolated from Jeddah coast of Red sea, Saudi Arabia. Saudi Journal of Biological Sciences 2014;21:57-64.
- Mathieson AC, Dawes CJ. Chapter 5 – Biology and ecology of Northwest Atlantic seaweeds. In: Konur O, ed. Handbook of Algal Science, Technology and Medicine: Academic Press, 2020:71-90.
- Gupta, S., Abu-Ghannam, N. (2011). Bioactive potential and possible health effects of edible brown seaweeds. Trends in Food Science & Technology, 22(6), 315–326.
- Abdel-Kareem MSM, ElSaied AAF. Chapter 2 – Global seaweeds diversity. In: El-Sheekh M, Abomohra AE-F, eds. Handbook of Algal Biofuels: Elsevier, 2022:39-55.
- Manivasagan P, Venkatesan J, Sivakumar K, Kim S-K. Pharmaceutically active secondary metabolites of marine actinobacteria. Microbiological Research 2014;169:262-278.
- Loh JT, Lam K-P. Fungal infections: Immune defense, immunotherapies and vaccines. Advanced Drug Delivery Reviews 2023;196:114775.
- Tesini BL, Dumyati G. Health Care-Associated Infections in Older Adults: Epidemiology and Prevention. Infectious Disease Clinics of North America 2023;37:65-86.
- Chu AL, Hickman M, Steel N, Jones PB, Davey Smith G, Khandaker GM. Inflammation and Depression: A Public Health Perspective. Brain, Behavior, and Immunity 2021;95:1-3.
- Cifaldi C, Ursu GM, D’Alba I, Paccoud O, Danion F, Lanternier F, Chiriaco M. Main human inborn errors of immunity leading to fungal infections. Clinical Microbiology and Infection 2022;28:1435-1440.
- Ali KA, Maity A, Roy SD, Das Pramanik S, Pratim Das P, Shaharyar MA. Chapter 4 – Insight into the mechanism of steroidal and non-steroidal anti-inflammatory drugs. In: Kazmi I, Karmakar S, Shaharyar MA, Afzal M, Al-Abbasi FA, eds. How Synthetic Drugs Work: Academic Press, 2023:61-94.
- Krienitz L. Archiv für Protistenkunde 1995;146:12.
- Chen X, Han M, Liang Y, Zhao W, Wu Y, Sun Y, Shao H, McMinn A, Zhu L, Wang M. Progress in ‘taxonomic sufficiency’ in aquatic biological investigations. Marine Pollution Bulletin 2022;185:114192.
- Alwaleed EA, Jillany A, Kasem NRA, Galal H. Evaluation of the pancreatoprotective effect of algal extracts on Alloxan-induced diabetic rat. Bioactive Carbohydrates and Dietary Fibre 2020;24:100237.
- Meena BR, Meena S, Chittora D, Sharma K. Antifungal efficacy of Thevetia peruviana leaf extract against Alternaria solani and characterization of novel inhibitory compounds by Gas Chromatography-Mass Spectrometry analysis. Biochemistry and Biophysics Reports 2021;25:100914.
- Schmid B, Coelho L, Schulze PSC, Pereira H, Santos T, Maia IB, Reis M, Varela J. Antifungal properties of aqueous microalgal extracts. Bioresource Technology Reports 2022;18:101096.
- Zohra M, Fawzia A. Hemolytic activity of different herbal extracts used in Algeria. Int J Pharm Sci Res 2014;5:495-500.
- Wise LE, Cannavacciulo R, Cravatt BF, Martin BF, Lichtman AH. Evaluation of fatty acid amides in the carrageenan-induced paw edema model. Neuropharmacology 2008;54:181-188.
- Guefack M-GF, Damen F, Ngaffo CMN, Kuete V. Acute and sub-chronic toxicities assessment of methanol bark extract of Hypericum roeperianum in rats. South African Journal of Botany 2022;150:691-701.
- Grizzle WE, Fredenburgh JL, Myers RB. 4 – Fixation of Tissues. In: Bancroft JD, Gamble M, eds. Theory and Practice of Histological Techniques (Sixth Edition). Edinburgh: Churchill Livingstone, 2008:53-74.
- Spencer LT, Bancroft JD. 6 – Tissue Processing. In: Bancroft JD, Gamble M, eds. Theory and Practice of Histological Techniques (Sixth Edition). Edinburgh: Churchill Livingstone, 2008:83-92.
- Rengasamy KRR, Mahomoodally MF, Aumeeruddy MZ, Zengin G, Xiao J, Kim DH. Bioactive compounds in seaweeds: An overview of their biological properties and safety. Food and Chemical Toxicology 2020;135:111013.
- Johnston CT, Aochi Y. Fourier transform infrared and Raman spectroscopy. Methods of Soil Analysis: Part 3 Chemical Methods 1996;5:269-321.
- Bedoux G, Hardouin K, Burlot AS, Bourgougnon N. Chapter Twelve – Bioactive Components from Seaweeds: Cosmetic Applications and Future Development. In: Bourgougnon N, ed. Advances in Botanical Research: Academic Press, 2014:345-378.
- Pradhan B, Bhuyan PP, Patra S, Nayak R, Behera PK, Behera C, Behera AK, Ki J-S, Jena M. Beneficial effects of seaweeds and seaweed-derived bioactive compounds: Current evidence and future prospective. Biocatalysis and Agricultural Biotechnology 2022;39:102242.
- Parthasarathy R, Chandrika M, Yashavantha Rao HC, Kamalraj S, Jayabaskaran C, Pugazhendhi A. Molecular profiling of marine endophytic fungi from green algae: Assessment of antibacterial and anticancer activities. Process Biochemistry 2020;96:11-20.
- Martens N, Schepers M, Zhan N, Leijten F, Voortman-Minderman G, Tiane A, Rombaut B, Poisquet J, Van De Sande N, Kerksiek A, Kuipers F, Jonker JW, Liu H, Luetjohann D, Vanmierlo T, Mulder MT. The use of seaweed-derived phytosterols to defeat Alzheimer’s Disease. Atherosclerosis 2022;355:6.
- Feng H, Jiang Y, Cao H, Shu Y, Yang X, Zhu D, Shao M. Chemical characteristics of the sesquiterpenes and diterpenes from Lauraceae family and their multifaceted health benefits: A review. Heliyon 2022;8:e12013.
- Pejin B, Ciric A, Glamoclija J, Nikolic M, Sokovic M. In vitro anti-quorum sensing activity of phytol. Natural Product Research 2015;29:374-377.
- Saha M, Bandyopadhyay PK. In vivo and in vitro antimicrobial activity of phytol, a diterpene molecule, isolated and characterized from Adhatoda vasica Nees. (Acanthaceae), to control severe bacterial disease of ornamental fish, Carassius auratus, caused by Bacillus licheniformis PKBMS16. Microbial Pathogenesis 2020;141:103977.
- Teleb WK, Tantawy MA, Osman NA, Abdel-Rahman MA, Hussein AA. Structural and cytotoxic characterization of the marine red algae Sarconema filiforme and Laurencia obtusa. Egyptian Journal of Aquatic Biology Fisheries 2022;26.
- Shahin A, Nabil-Adam A, Elnagar K, Osman H, Shreadah MA. Bioactivity and metabolomics fingerprinting characterization of different organic solvents extracts of Padina pavonica collected from Abu Qir Bay, Egypt. Egyptian Journal of Chemistry 2022;65:207-225.
- Ramu Ganesan A, Subramani K, Shanmugam M, Seedevi P, Park S, Alfarhan AH, Rajagopal R, Balasubramanian B. A comparison of nutritional value of underexploited edible seaweeds with recommended dietary allowances. Journal of King Saud University – Science 2020;32:1206-1211.
- Bordoloi M, Saikia S, Bordoloi PK, Kolita B, Dutta PP, Bhuyan PD, Dutta SC, Rao PG. Isolation, characterization and antifungal activity of very long chain alkane derivatives from Cinnamomum obtusifolium, Elaeocarpus lanceifolius and Baccaurea sapida. Journal of Molecular Structure 2017;1142:200-210.
- Rushdi MI, Abdel-Rahman IAM, Saber H, Attia EZ, Madkour HA, Abdelmohsen UR. A review on the pharmacological potential of the genus Padina. South African Journal of Botany 2021;141:37-48.
- Maghawri A, Marzouk SS, Ezz El-Din HM, Nashaat M. Effect of brown algae Padina pavonica as a dietary supplement on growth performance and health status of cultured Oreochromis niloticus. The Egyptian Journal of Aquatic Research 2023;49:379-385.
- Abu-Ghannam N, Rajauria G. 8 – Antimicrobial activity of compounds isolated from algae. In: Domínguez H, ed. Functional Ingredients from Algae for Foods and Nutraceuticals: Woodhead Publishing, 2013:287-306.
- Al-Enazi NM, Awaad AS, Zain ME, Alqasoumi SI. Antimicrobial, antioxidant and anticancer activities of Laurencia catarinensis, Laurencia majuscula and Padina pavonica extracts. Saudi Pharmaceutical Journal 2018;26:44-52.
- Chiao-Wei C, Siew-Ling H, Ching-Lee W. Antibacterial activity of Sargassum polycystum C. Agardh and Padina australis Hauck (phaeophyceae). African Journal of Biotechnology 2011;10:14125-14131.
- Xiong N-X, Luo S-W, Fan L-F, Mao Z-W, Luo K-K, Liu S-J, Wu C, Hu F-Z, Wang S, Wen M, Liu Q-F. Comparative analysis of erythrocyte hemolysis, plasma parameters and metabolic features in red crucian carp (Carassius auratus red var) and triploid hybrid fish following Aeromonas hydrophila challenge. Fish & Shellfish Immunology 2021;118:369-384.
- Thirunavukkarasu R, Pushparaj S, Arokiarajan MS, Pandi P, Subramanian K, Joseph J. Chapter 17 – Microbial symbionts from Algae. In: Dharumadurai D, ed. Microbial Symbionts: Academic Press, 2023:311-326.
- Rivera Rodríguez R, Johnson JJ. Terpenes: Modulating anti-inflammatory signaling in inflammatory bowel disease. Pharmacology & Therapeutics 2023;248:108456.
- Nabti B, Bammoune N, Meliani H, Stambouli B. Antioxidant and antimicrobial activities of Spirulina from the region of Tamanrasset, Algeria. Journal of Herbal Medicine 2023;41:100748.
- Rukshala D, de Silva ED, Ranaweera BVLR, Fernando N, Handunnetti SM. Anti-inflammatory effect of leaves of Vernonia zeylanica in lipopolysaccharide-stimulated RAW 264.7 macrophages and carrageenan-induced rat paw-edema model. Journal of Ethnopharmacology 2021;274:114030.
- Meng X, Li L, Huang C, Shi K, Zeng Q, Wen C, Grad S, Alini M, Qin L, Wang X. Anti-inflammatory and anabolic biphasic scaffold facilitates osteochondral tissue regeneration in osteoarthritic joints. Journal of Materials Science & Technology 2023;156:20-31.
- Rother K, Rother U, Hänsch G. The Role of Complement in Inflammation. Pathology – Research and Practice 1985;180:117-124.
- Manikandan R, Parimalanandhini D, Mahalakshmi K, Beulaja M, Arumugam M, Janarthanan S, Palanisamy S, You S, Prabhu NM. Studies on isolation, characterization of fucoidan from brown algae Turbinaria decurrens and evaluation of it’s in vivo and in vitro anti-inflammatory activities. International Journal of Biological Macromolecules 2020;160:1263-1276.
- Youn GS, Lee KW, Choi SY, Park J. Overexpression of HDAC6 induces pro-inflammatory responses by regulating ROS-MAPK-NF-κB/AP-1 signaling pathways in macrophages. Free Radical Biology and Medicine 2016;97:14-23.
- Sharma PP, Chonche MJ, Mudhol S, Muthukumar SP, Baskaran V. Anti-inflammatory efficacy of brown seaweed (Padina tetrastromatica) in 3T3-L1 adipocytes and low-dose LPS induced inflammation in C57BL6 mice. Algal Research 2023;71:103027.
- Araico A, Terencio MC, Alcaraz MJ, Domínguez JN, León C, Ferrándiz ML. Evaluation of the anti-inflammatory and analgesic activity of Me-UCH9, a dual cyclooxygenase-2/5-lipoxygenase inhibitor. Life Sciences 2007;80:2108-2117.