

Titilola Oyenike Animashaun1, Morenike Olutunmbi Adeoye-Isijola1, Kubendran Kista Naidoo2
, Roger Murugas Coopoosamy2
, Olufunmiso Olusola Olajuyigbe1,2*
1Department of Microbiology, School of Science Technology, PMB 4005, Babcock University, 121103, Ilisan Remo, Ogun State, Nigeria
2Department of Nature Conservation, Mangosuthu University of Technology, Durban, KwaZulu-Natal, South Africa
Corresponding Author E-mail: funmijuyigbe12@yahoo.com
DOI : https://dx.doi.org/10.13005/bpj/2687
Abstract
In Nigeria, urinary tract infections (UTIs) are amid the most prevalent bacterial contagions, with a higher incidence rate in women. Depending on the severity of the infection, different antibiotics are used to treat UTIs. Herbal remedies have, on the other hand, been seen as the paranormal means of treating UTIs while antibiotic resistance has emerged as a problem for medics. This study examined the methanol extract of Rhaphiostylis beninensis (MRB) for its phytochemical and bioactive components, antioxidant, and antibacterial activities. From the study, the methanol extract's antioxidant activities grew stronger as concentration increased. Alkaloids, saponin, tannins, cardiac glycosides, flavonoids, and reducing sugar—giving the extract its pharmacological potential—were among the phytochemicals identified from the phytochemical screening. The GC-MS Chromatogram showed that there are 42 bioactive compounds in the MRB with the most prominent compounds being (Z,Z)-9,12-Octadecadienoic acid 19.96%, Lupeol 18.96%, cis-13-Octadecenoic acid 9.96%, n-Hexadecanoic acid 9.87%, 3',5'-Dimethoxyacetophenone 6.67%, 4-((1E)-3-Hydroxy-1-propenyl)-2-methoxyphenol 3.21%, Octadecanoic acid 2.98%, Capsaicin 2.58%, α-Amyrin 2.53%, 9-Octadecenoic acid (Z)-, 2,3-dihydroxypropyl ester 2.49%, and Vitamin E 2.45%. The MRB showed significant minimum inhibitory concentration values against Pseudomonas putida PA25T, Escherichia coli EC3AT, Pseudomonas aeruginosa PA129AT and Serratia marcescens and a significant MBC value against Escherichia coli EC3AT. This study emphasizes the medicinal efficacy of R. beninensis root and indicated that the plant could make a promising candidate for the isolation of bioactive compounds that could be synthesized as medications for the treatment of bacteria in uropathogenic disorders.
Keywords
Antibiotics; Antioxidant; Bioactive Compounds; GC-MS Analysis; Phytochemicals; Urinary tract infections
Download this article as:
Copy the following to cite this article: Animashaun T. Q, Isijola M. O. A, Naidoo K. K, Coopoosamy R. M, Olajuyigbe O. O. Gas Chromatography-Mass Spectrometry Analysis, Phytochemical Screening, Antioxidant and Antibacterial Activity of Methanol Root Extract of Rhaphiostylis beninensis (Hook.f.) Planch. [family ICACINACEAE] against Uropathogens. Biomed Pharmacol J 2023;16(2). |
Copy the following to cite this URL: Animashaun T. Q, Isijola M. O. A, Naidoo K. K, Coopoosamy R. M, Olajuyigbe O. O. Gas Chromatography-Mass Spectrometry Analysis, Phytochemical Screening, Antioxidant and Antibacterial Activity of Methanol Root Extract of Rhaphiostylis beninensis (Hook.f.) Planch. [family ICACINACEAE] against Uropathogens. Biomed Pharmacol J 2023;16(2). Available from: https://bit.ly/3XDFQhF |
Introduction
One of the most ubiquitous bacterial illnesses worldwide is urinary tract infections (UTIs). About 50–60% of women may experience UTIs at some point in their lives, making it a predominant bacterial infection responsible for close to 25% of all infections in women. About 150 million cases per year, as an estimate, was indicated in 2016. Although both men and women can become seriously ill from these diseases, women are more likely to contract them1. Women who are sexually active, especially those who use spermicidal products and diaphragms for contraception, are more likely to develop UTIs due to immune suppression in such females, even though the spread of these infections to the kidney is more proliferating in pregnant women owing to their enfeebled immune systems in gestation. While UTIs in pregnant women may result in early labor and high blood pressure2, post-menopausal women experience higher rates of UTIs because of the pelvic prolapse, deficiency of estrogen, amplified peri-urethral colonization by Escherichia coli, diabetes mellitus, and reduction of Lactobacilli spp. amongst the vaginal flora3.
Although Staphylococcus spp., Proteus vulgaris and Pseudomonas spp. are infrequent bacteria responsible for UTIs4, Proteus mirabilis, Enterobacter cloacae, Enterobacter aerogenes, Pseudomonas aeruginosa, Proteus vulgaris, Staphylococcus aureus, Escherichia coli and Klebsiella pneumoniae have been identified as the causative agents of UTIs5,6. While E. coli and K. pneumoniae are the most common uropathogens and UTI indicator organisms, respectively, according to the World Health Organization’s Global Antimicrobial Surveillance System (GLASS)7,8, over and above 80% of all unsophisticated community acquired urinary tract infections (CA-UTIs) are triggered by E. coli alone9. In general, E. coli in the intestine are usually normal flora and not detrimental, but if it gets to the vaginal hole during washing or after sexual activity, it could cause infections on entering and colonizing the bladder. For instance, , some E. coli pathotypes such as uropathogenic E. coli (UPEC) in immunocompromised individuals have developed enabling capabilities to breach the characteristically sterile urinary system and cause both symptomatic and asymptomatic infections9. Numerous virulence factors, including polysaccharide capsule, lipopolysaccharide (LPS), flagella, pili, non-pilus adhesins, outer-membrane proteins, outer-membrane vesicles, secreted toxins and TonB-dependent iron-uptake receptors such as siderophore receptors are used to colonize the bladder by this organism. All of these elements offer great potential for the establishment of treatments and vaccines10,11. Amphipathic molecules called LPS are composed of lipid A or endotoxin, core phosphorylated oligosaccharide and a lengthy side chain known as O antigen12. LPS structural components, rapid bladder colonization, reservoir formation, and induction of innate and adaptive immune responses have roles to play in facilitating various phases of the UPEC life cycle13. When there is a decrease in the quantity of LPS at the surface of the cell, LPS confers resistance to hydrophobic antibiotics and hypersensitivsity to hydrophobic harmful molecules such as bile salts and certain antibiotics14.
Since many of the agents causing UTIs have developed resistance to majority of the antibiotics used to treat them15,16, diverse guidelines for treating UTIs have been adopted in innumerable regions of the world10 based on noteworthy scientific evidence. According to Magyar et al.15, resistance of E. coli to ciprofloxacin increased remarkably from 19% to 25%, resistance of K. pneumoniae to cephalosporins was 60%, and the percentage of carbapenem-resistant P. aeruginosa also increased noticeably. While multidrug-resistant pathogens are increasingly responsible for UTIsworldwide17, antibacterial resistance has led to higher rates of morbidity and mortality, higher resource use, increased costs, decreased hospital activity, and antibiotic treatment guidelines that favor broad-spectrum empiric therapy18,19. A great impediment to using these empirical therapy options is provided by the rise in antibiotic resistance20. Due to the fact that herbal medications are secure, affordable, and simple to use, concentrated attention has been directed to the therapeutic potential of medicinal plants2. Consequently, medicinal plants have been widely investigated21, and various essential chemical constituents with tremendous therapeutic potential have been identified to indicate their pharmacological properties22-24. While antimicrobial, anti-inflammatory, and antioxidant activities of plant extracts have been documented25,26, a wide variety of their chemical components have therapeutic potentials in vitro27,28. Since there are no credible scientific data indicating that bacteria have evolved a resistance to therapeutic plants, these phytochemicals could serve as the basis for the manufacture of secure ground-breaking medications for treating infections and diseases. Thus, by using these natural medications, the issue of resistance resulting from the usage of conventional treatment might be resolved.29.
Rhaphiostylis beninensis Planch ex Benth (Icacinaceae) is a woody climber with a wide variety of curative and culinary uses. It grows in the West African subcontinent and South-Western Nigeria. It goes by many different names in Nigeria depending on one’s location and its usage. Atapata (Yoruba), osumadin (Benin), kpolokoto (Ibos), umeni (Urhobos), and kumeni (Itsekiris) are all names for the same plant. R. beninensis belongs to the Icacinaceae family, which contains a variety of bioactive phytochemicals30. Innumerable pharmacological and biological properties of the plant’s root extract have also been reported. Antibacterial, analgesic, and anti-inflammatory properties have been reported for the plant31. The leaf and root are used in folkloric treatment of arthritis, rheumatism, skin illnesses, mental disorders, convulsions, and eye problems, while the leaf decoction is used as a mouthwash and a sore wash32,33. Adjanohoun et al.34 reported mosquito repellent activities of its aqueous leaf extract. Gram-positive and Gram-negative bacteria as well as fungus were found to be resistant to the antibacterial activities of the oil extracted from the root, stem bark extracts, and fruit35. While anthraquinones, flavonoids, and triterpenes were discovered in the plant36 and a thiourea derivative, N, N-di (4-methyoxybenzyl) thiourea, with anti-inflammatory activity has been isolated from the root of the plant37, information on the chemis try, pharmacology, and antibacterial potential of oils produced from the methanol extract of R. beninensis roots is minimal. So, in response to its folkloric use, this study investigated the antioxidant, and antibacterial activities of the methanol extract of R. beninensis against uropathogenic bacteria as well as identify bioactive phytochemicals crucial in developing novel drugs from natural products.
Materials and Methods
Collection of Plant materials
The roots of Rhaphiostylis beninensis were harvested from its natural habitat in Ijebu-Ode, Ogun State, Nigeria, rinsed with sterile distilled water and allowed to air-dry. The roots were identified and deposited at the Nigerian Forestry Herbarium in Ibadan (FHI) under voucher number FHI 113354.
Plant Extraction
The extraction was done using the method of Adeshina et al.38. The roots were pulverized before being sealed up in an airtight container free of grease. Four hundred grams of the sample was soaked in 1000 mL of methanol, left to stand for 72 h while being stirred frequently, sieved with three folds sterile muslin cloth and then filtered with Whatman No. 1 filter paper29. The extracting solvent was effectively evaporated from samples with a rotary evaporator at 68°C. The extract was properly stored pending additional analyses39.
Qualitative Phytochemical analysis
Using standard methods outlined by Sofowora40 and Trease & Evans41, the methanol extract was examined for the occurrence of several phytochemicals.
Test for Saponins
The extract was mixed briskly with 20 mL of water in a graduated measuring cylinder to dilute it, and the concoction was allowed to stand for 15 min to test for the presence of saponin. It was clear that saponin was present because foam was formed. The foam height (H2-H1) was then determined.
Test for Alkaloids
A percentage of the extract was dispensed in a test tube, along with ethanol and diluted hydrochloric (HCl) acid (50:50). The concoction was heated in boiling water for 10 min before filtering to check for alkaloids. Mayer’s Test: When Mayer’s reagent (1.36 g of mercuric chloride and 5.00 g of potassium iodide were mixed in 100 mL of water) was applied to the filtrate, a buff-white yellow precipitate formed, indicating the occurrence of alkaloids.
Test for Tannins
A beaker containing 0.30 g of the methanol extract was boiled in a water bath for 10 min to test for tannins. Boiling was followed by filtering with Whatman number 42 (125 mm) filter paper. Then, 3 drops of 1% ferric chloride were added to 5 mL of the filtrate. The presence of tannins was suggested by the development of brownish-green or blue-black coloring.
Test for Steroids
To test for steroids, chloroform (3 mL) was added to methanol extract (5 mg) before filtering into test-tube. The test-tube was tilted and concentrated Sulphuric acid (H2SO4)(2 mL) was carefully added through the side of the test-tube. The presence of brown and reddish color at chloroform phase showed existence of steroid.
Test for Terpenoids
For the terpenoids test, 0.30 g of the methanol extract was extracted for 2 h in a beaker with sterile distilled water (30 mL). To partition the aliquot, the extract (5 mL) was added to chloroform (2 mL) combined with concentrated H2SO4 (3 mL). Reddish brown coloring at the border line confirmed the existence of terpenoids.
Test for Phenols
For phenols test, 2 mL of1% FeCl3 was mixed with the extract.The presence of blue-black (violet) or blue green coloration confirmed the availability of phenol.
Test for Phlobatannins
To test for phlobatannins, sterile distilled water (30 mL) was added to 0.30 g of powdered sample in a beaker. After extracting for 24 h, the aqueous extract (10 mL) was boiled with 1% aqueous hydrochloric acid (5 mL). Red precipitate indicated the presence of phlobatamins.
Test for Flavonoids
To test for flavonoids, 0.30 g of powdered plant material was extracted for 2 h with 30 mL of distilled water before filtering with Whatman filter paper number 42 (125 mm). Five milliliters of 1.0 M diluted ammonia solution were added to 10 mL of the aqueous filtrate of extract. This was followed by adding 5 mL of H2SO4. The presence of yellow colour that vanished upon standing indicated the occurrence of flavonoids.
Test for Reducing Sugars
A test tube containing 2 mL of aqueous extract and 1 mL of each of Fehling solutions A and Solution B was boiled for 10 min to check for reducing sugars. The yellow or brick-red precipitate produced indicated occurrence of reducing sugar.
Test for Glycosides
In this test, 20 mL of water and 2 g of the extract were combined, heated for 5 min in a water bath and then filtered through Whatman number 42 filter paper (125 mm). To detect glycosides, two tests were run with the filtrate. First, each of Fehling’s solution A and solution B (0.2 mL) were mixed with 5 mL of the filtrate until an alkaline mixture was obtained. Positive results were seen when brick-red coloring was present on heating. Second, the aforesaid test was repeated using 15 mL of 1.0 M H2SO4 instead of water, and the quantity of precipitate obtained was compared to those obtained from the initial test. The presence of glycosides was confirmed by high precipitate content and low precipitate content confirmed the nonexistence of glycosides.
Phytochemical analysis
Quantitative determination of flavonoid concentration
The total flavonoid content of the methanol extract of R. beninensis was determines as described by Ejikeme et al.42. Fifty milliliters of 80% aliquot were added to 2.50 g of the plant material in a 250 mL beaker. The mixture was covered and allowed to stand for 24 h at room temperature. The extraction was repeated three consecutive times by adding the same volume of methanol after removing the supernatant. The mixture was filtered before transferring to a crucible and dried in a water bath. The crucible and its content were weighed after being cooled down in a desiccator.

Quantitative determination of alkaloid concentrations
The alkaloid was determined quantitatively as described by Ezeonu & Ejikeme43. The extract (2.50 g) was liquefied in 200 mL of 10% acetic acid in methanol, and permitted to stand for 4 h. The filtrate was concentrated in a water bath to a quarter of its initial volume before 15 drops of concentrated ammonium hydroxide (NH4OH) was added until a precipitate was formed. After 3 h of sedimentation, the supernatant was removed by filtration, and the precipitate was washed with 20 mL of 0.1 M ammonium hydroxide before filtering. After drying the residue in the oven, the percentage of alkaloid was estimated.

Quantitative determination of total saponin
The saponin was determined quantitatively as described by Ejikeme et al.42 and Obadoni & Ochuko44. Here, 5 g of powdered sample was added to 100 mL of aqueous ethanol (20%) in a conical flask (250 mL)before being heated with constant stirring at a temperature of 55oC in a water bath for 4 h. After filtration, the procedure was repeated two more times. The extract was vaporized to 40 mL at 90oC in a water bath. In a separating funnel, diethyl ether (20 mL) was added to the concentrate before shaken strongly. The aqueous layer was recovered and the ether layer was discarded. This purification process was repeated twice. n-butanol (60 mL) was added to the aqueous layer recovered before extracting twice with 10 mL of 5% sodium chloride. The residual solution was heated in a water bath for 30 min after discarding the sodium chloride layer, transferred to a crucible and dried in an oven to a constant weight.
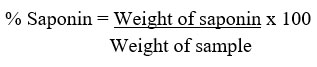
Quantitative determination of total tannins
The tannin content of the methanol extract of R. beninensis was determined as described by Ejikeme et al.42 and Amadi et al.45. Folin-Denis reagent was prepared by liquefying sodium tungstate (Na2WO4) (50 g) in distilled water (37 mL) while phosphomolybdic acid (H3PMo12O40) (10 g) and orthophosphoric acid (H3PO4) (25 mL) were added to this reagent. This mixture was refluxed for 2 h, cooled, and made up to 500 mL with distilled water. The extract (1 g) was added to distilled water (100 mL) in a conical flask, boiled for 1 h on an electric hot plate and filtered using Whatman number 42 (125 mm) filter paper in a volumetric flask (100 mL). Folin-Denis reagent (5 mL), saturated Na2CO3 (10 mL) solution, diluted extract (10 mL) and distilled water (50 mL) were added together in a conical flask (100 mL) for a change in colour. After constant stirring, the aliquot stood for 30 min in a water bath at 25°C before determining the optical density at 700 nm using a UV/VIS spectrophotometer, and its results were compared to a typical tannic standard curve obtained by dissolving 0.20 g of tannic acid in distilled water before being diluted up to 200 mL mark (1 mg/mL). Five different test tubes were filled with varied amounts of the standard tannic acid solution (0.2-1.0 mg/mL), followed by adding Folin-Denis reagent (5 mL), saturated Na2CO3 (10 mL), and distilled water to bring the total volume to 100 mL. The solution was allowed to stand for 30 min in a water bath at 25oC. Optical density (absorbance) versus tannic acid concentration was plotted on a graph.

where 𝐶 is concentration of tannic acid read off the graph.
Quantitative determination of total phenolic concentration
Quantitative determination of phenol was carried out as described by Ezeonu & Ejikeme43. Two grams of powdered sample were defatted for 2 h in ether (100 mL) using a soxhlet apparatus. The defatted sample (0.50 g) was boiled in ether (50 mL) for 15 min to extract the phenolic compounds. For colour development, distilled water (10 mL), 0.1 N ammonium hydroxide (2 mL) solution and concentrated amyl alcohol (5 ml) were added to the extract (5 mL) and left to react for 30 min. The optical density was measured at 505 nm while tannic acid (0.20 g) was dissolved in distilled water (200 mL) mark (1 mg/mL) to prepare the phenol standard curve. Varied concentrations (0.2–1.0 mg/mL) of the standard tannic acid solution were pipetted into five different test tubes to which NH4OH (2 mL), amyl alcohol (5 mL), and water (10 mL) were added for colour development after reacting for 30 min. The optical density was measured with a UV/VIS TG 50 spectrophotometer at 505 nm.
GC-MS Quantification
GC–MS is one of the best, fast and most accurate approach to detect various compounds including alcohols, nitro compounds, alkaloids, organic acids, steroids, long chain hydrocarbons, esters and amino acids, and requires little amount of plant extracts. In this study, the GC–MS analysis detected and identified the chemical compounds present in R. beninensis as described by Fagbemi et al.24.
Determination of antioxidant Activity by DPPH Radical Scavenging Activity Assay
The DPPH radical scavenging assay, as described by Chen et al.46, was used to examine the extract’s capacity to scavenge free radicals. Briefly, 0.1 mM DPPH (1 mL) in methanol was thoroughly mixed with extract (1 mL) in methanol at different concentrations (25–100 μg/mL), allowed to stand for 30 min at room temperature before measuring the absorbance with a UV-Vis Spectrophotometer at 517 nm. The decreased absorbance in the reaction mixture indicated a higher free radical scavenging capacity. DPPH scavenging effect’s percentage inhibition was calculated using the equation:
DPPH Scavenging effect (%) = [(A0-A1)/A0] x 100
Where A0 was the absorbance of the control and A1 was the absorbance in the presence of the standard sample or extract. The IC50 value represented the concentration of the extract that inhibited production of DPPH activities by 50%. The experiment was repeated three times at different concentrations.
Determination of Antioxidant Activity by Ferric Reducing Antioxidant Power Assay (FRAP)
Using Otang et al.47 approach, the ferrous reducing antioxidant capacity of the extract was assessed. One milliliter of the extract (25-100 µg/mL) was mixed with 0.1 M sodium phosphate buffer (2.5 mL) (pH 6.6) and 1% w/v potassium ferrocyanate (2.5 mL) [K3Fe(CN)6] in a 250 ml conical flask before incubating at 50°C for 20 min. Following this, trichloroacetic acid (2.5 mL) (10% w/v) was added to the mixture before centrifuging at 5000 rpm for 10 min. The upper layer (5 ml) was mixed with of fresh FeCl3 (0.1%, w/; 0.5 mL), and the absorbance was measured at 700 nm in comparison to a blank. Gallic acid was used as the control. The percentage inhibition in FRAP was calculated using the following equation:
FRAP percentage inhibition (%) = [(A0-A1)/A0] x 100
where A0 was the absorbance of the control and A1 was the absorbance in the presence of the standard sample or extract. All tests were performed in triplicate. Higher absorbance of the reaction mixture indicated great reducing power.
Determination of Antioxidant Activity by Nitric Oxide Radical Scavenging Assay
The nitric oxide radical scavenging activity was evaluated as previously described by Silva and Soysa48. Different concentrations (25–100 µg/mL) of the extract and the standard ascorbic acid were prepared by serially diluting them with distilled water. These were kept for subsequent use and stored at 4oC. Griess reagent was prepared by mixing equal volumes of sulphanilamide (1%) in phosphoric acid (2.5%) and naphthylethylene diamine dihydrochloride (0.1%) in phosphoric acid (2.5%) proximately before use. Following this, sodium nitroprusside (10 mM) in phosphate buffered saline (0.5 mL) was mixed with 1 mL of the different concentrations of the methanol extract (25−100 µg/mL) and incubated at 25oC for 180 min. The extract and the ascorbic acid used as control were mixed with an equivalent volume of recently prepared Griess reagent. The tubes used as control contained the same concentrations of methanol extract without sodium nitroprusside while 150 µL of the reaction mixture was transferred to a 96-well plate. Using a UV/VIS TG 50 Plus UV-VIS microplate reader, the absorbance at 546 nm was determined (Molecular Devices, GA, USA). The percentage inhibition of the extract and the standard was calculated and recorded. The inhibition percentage of the nitrite radical scavenging activity of both methanol extract and ascorbic acid were calculated using the following formula:

Where 𝐴control = absorbance of the control sample and 𝐴test = absorbance in the presence of the extract or standard. The experiment was conducted three times at different concentrations.
Determination of Antioxidant Activity by Lipid Peroxidation Assay
The lipid peroxidation assay was valued as described by Murugan & Parimelazhagan49. Ten microliters of extract and a standard solution containing 1,1,3,3-tetramethoxypropane (TEP) at varying concentrations of 25, 50, 75 and 100 μg/mL and phosphate buffer (20 mM; 40 μL) (pH 7.0) were added to test tubes in an ice bath. In a tightly sealed test tube, sodium dodecyl sulfate (SDS) (3%; 50 μL), HCl (0.1 N; 200 μL), phosphotungstic acid (10%; 30 μL) and 2-thiobarbituric acid (TBA) (0.7%;100 μL) were combined and boiled at 100°C for 30 min in a water bath. The reaction mixture was mixed with n-butanol (400 μL) and then centrifuged at 3000 rpm for 10 min. Supernatants were collected and pass through a UV/VIS spectrophotometer at a wavelengths of 515/555 nm.

Where 𝐴control = absorbance of the control sample and 𝐴test = absorbance in the presence of the extract or standard. The experiment was conducted three times at each of the concentrations.
Determination of Total Antioxidant Capacity
Total antioxidant capacity (TAC) of the sample was evaluated as indicated by Kattamis et al.50. Aliquots (100 µL) of KMnO4 solution (5 mmol/L) made by dissolving 79 mg of KMnO4 in distilled water (100 mL) were added to the extract and evenly mixed by shaking. After warming for 30 min in a water bath at 37°C, the mixture’s absorbance was measured using a UV/VIS spectrophotometer (optical density, OD, of 570 nm).

Where 𝐴control = absorbance of the control sample and 𝐴test = absorbance in the presence of the samples of extracts or standards. The experiment was conducted three times at each of the concentrations.
Antimicrobial activity
Bacterial isolates used in this study included Pseudomonas putida PA25T, Pseudomonas aeruginosa PA129T, Pseudomonas aeruginosa PA29T, Morganella morganii PA17T, Serratia marcescens PA18T, Klebsiella pneumoniae KB24AT, Proteus mirabilis EC28T, Escherichia coli EC3AT, Acinetobacter indicus KB45AT and Acinetobacter indicus EC41TT isolated from the urinary tract infection of patients at Olabisi Onabanjo University Teaching Hospital, Sagamu, Ogun State, Nigeria, were used for sample collections between June and October, 2017. The ethical approval to carry out the study was granted with a certificate indicating National code for Health Research Ethics NHREC/24/01/2020 and Babcock University Health Research Ethics Committee BUHREC 651/21 number from Babcock University, Ilisan Remo, Ogun State, Nigeria. Susceptibility of the uropathogens to the methanol extract of R. beninensis was evaluated by determining the minimum inhibitory concentrations (MICs) and minimum bactericidal concentrations (MBCs) of the extract with macrobroth dilution methods26.
Determination of minimum inhibitory concentration (MIC) of the extracts
Minimum inhibitory concentrations (MICs) of the extract, defined as the lowest concentrations maintaining or reducing of inoculums viability51, was determined by serial tube dilution technique26 against the bacterial isolates. For antibacterial assay, concentrations of extract that ranged between 20 µg/mL and 10,000 µg/mL and those of erythromycin used as positive control ranged between 0.0122 µg/mL and 50 µg/mL were prepared by serial dilutions in tubes containing double strength Mueller Hinton broth and inoculated with 100 µL of each of the bacterial isolates. Blank broth of Mueller Hinton was used as negative control.
Determination of minimum bactericidal concentration (MBC) of the extracts
For the MBC assay, one loopful of culture from each of the first five broth cultures without bacterial growth in the MIC tubes was inoculated into fresh nutrient agar plates26 and incubated at 37°C for 24 h. After the incubation periods, the lowest concentration of the extract that showed no sign of bacterial growth on the solid medium was regarded as the MBC value for this extract. This finding was supported by the MIC test tube, which showed no growth after 48 h of the incubation period.
Results
The qualitative phytochemical analysis of the methanol extract of R. beninensis indicated alkaloids, saponin, tannins, cardiac glycosides, flavonoids and reducing sugar which are of pharmacological importance (Table 1).
Table 1: Qualitatively determined phytochemicals in the methanol extract of R. beninensis
Phytochemical compounds |
Methanolic extract |
Saponins |
+ |
Tannins |
+ |
Phlobatannins |
– |
Flavonoids |
+ |
Cardiac Glycosides |
+ |
Alkaloid |
+ |
Reducing Sugar |
+ |
Terpenoid |
+ |
Phenol |
+ |
Steroids |
– |
Quantitative phytochemical analysis
The quantitative phytochemical analysis of the methanol extract of R. beninensis in Table 2 showed that the total reducing sugar content was 31.27±0.08 mg/g, alkaloid content was 28.99±0.03 mg/g, terpenoid content was 25.96±0.06 mg/g and saponin content was 22.73±0.19 mg/g while those of tannins and phlobatannins in the extract were 12.39±0.04 and 6.73±0.07 mg/g, respectively. However, the cardiac glycoside, and steroid content were absent.
Table 2: Quantitatively determined phytochemicals in the methanol extract of R. beninensis
Phytochemical compounds |
MRB (mg/100g) |
Saponins |
22.73 ± 0.19 |
Tannins |
12.39 ± 0.04 |
Phlobatannin |
6.73 ± 0.07 |
Cardiac Glycosides |
0 |
Alkaloid |
28.99 ± 0.03 |
Reducing Sugar |
31.27 ± 0.08 |
Terpenoid |
25.96 ± 0.06 |
Steroids |
0 |
Gas Chromatography Mass Spectrometry (GCMS) Analysis
Figure 1 shows the GCMS chromatogram of the chemical compounds in the extract of R. beninensis. The GCMS analysis showed 42 chemical compounds in the methanol (MRB) extract of R. beninensis. The name, chemical structure and pharmacological activities of each of the chemical compounds in the methanol extract are presented in Table 3. The most notable compounds with chemical composition’s percentages ≥ 1.0% are (Z,Z)-9,12-Octadecadienoic acid (19.96%), Lupeol (18.96%), cis-13-Octadecenoic acid (9.96%), n-Hexadecanoic acid (9.87%), 3′,5′-Dimethoxyacetophenone (6.67%), 4-((1E)-3-Hydroxy-1-propenyl)-2-methoxyphenol (3.21%), Octadecanoic acid (2.98%), Capsaicin (2.58%), α-Amyrin (2.53%), 9-Octadecenoic acid (Z)-, 2,3-dihydroxypropyl ester (2.49%), Vitamin E (2.45%), Chondrillasterol (1.98%), Gingerol (1.70%), Dihydrocapsaicin (1.53%), (Z,Z)-9,12-Octadecadienoic acid, 2,3-dihydroxypropyl ester (1.45%), 2,6-dimethoxy-4-(2-propenyl)-phenol (1.23%), 4,7-Dimethoxy-2-methylindan-1-one (1.38%), Hexadecanoic acid, and 2-hydroxy-1-(hydroxymethyl)ethyl ester 1.15%. From the literature search, most of these bioactive compounds exhibited varied pharmacological activities and other therapeutic potentials except 3′,5′-Dimethoxyacetophenone, 4-amino-2-ethyl-6-methyl-3-Pyridinol, Benzene, ethenylpentaethyl-, 2-Allyl-1,4-dimethoxy-3-vinyloxymethylbenzene Benzene, hexaethyl- and Benzene, hexaethyl-, having no biological activities reported in literatures.
![]() |
Figure 1: Chromatogram of the GC-MS analysis of methanol extract of Rhaphiostylis beninensis |
![]() |
Table 3: GC-MS Analysis of the bioactive compounds of methanol extract of R. beninensis (MRB) |
DPPH radical scavenging activity
In this study, the radical scavenging activity of the methanol extract of R. beninensis was concentration-dependent. The capacity of the extract to mop up DPPH radicals increases as its concentration increases as shown in Figure 2. At all the studied concentrations, the extract produced a noticeably high DPPH radical scavenging action. Ascorbic acid, however,, used as control showed higher radical scavenging action and an IC50 value of 28.42 µg/mL compared with that of the extract having a higher IC50 value of 42.19 µg/mL.
![]() |
Figure 2: DPPH radical scavenging activity (%) (Mean ± Standard deviation) of methanol extract of R. beninensis. Key: MRB = Extract with Methanol, AA= Ascorbic acid used as control. |
Ferric reducing power antioxidant assay (FRAP)
Figure 3 illustrates the dependence of the reducing power of the methanol extract of R. beninensis (25–100 µg/mL) on concentration. As concentrations increased, so did the extract’s FRAP activity. At all the studied concentrations, the methanol extract of R. beninensis produced a considerably high FRAP activity. The ascorbic acid was used as control and it showed FRAP activity and an IC50 value of 77.29 µg/mL in comparison with the methanol extract of R. beninensis having an IC50 value of 332 µg/mL.
![]() |
Figure 3: Ferric reducing antioxidant power (FRAP) activity of methanol extract of R. beninensis. Key: MRB = Extract with Methanol, AA= Ascorbic acid. |
Nitric oxide radical scavenging assay
The nitric oxide scavenging effect of the methanol extract of R. beninensis (25–100 µg/mL) was concentration-dependent. The effects of the methanol extract’s nitric oxide scavenging activity at various doses were depicted in Figure 4. Nitric oxide scavenging effets for R. beninensis had an IC50 value of 41.29 µg/mL, whereas ascorbic acid demonstrated potent nitric oxide inhibition with an IC50 value of 34.13 µg/mL.
![]() |
Figure 4: Nitric oxide radical scavenging activity of methanol extract of R. beninensis. Key: MRB = Extract with Methanol, AA= Ascorbic acid used as control. |
Lipid peroxidation assay
As demonstrated in Figure 5, concentration has an effect on the lipid peroxidation assay of the methanol extract of R. beninensis (25–100 µg/mL). In this investigation, the concentration of the plant extract that can scavenge 50% of the lipid peroxidation radicals (IC50) was established. The results indicated that the IC50 value for the methanol extract of R. beninensis was 59.18 µg/mL as opposed to 33.44 µg/mL for the ascorbic acid used as standard.
![]() |
Figure 5: Lipid peroxidation activity of methanol extract of R. beninensis. Key: MRB = Extract with Methanol, AA= Ascorbic acid used as control. |
Total antioxidant capacity
Table 4 showed methanol extract of R. beninensis has a higher total antioxidant capacity (TAC) content (42.11 ± 1.13 mg/100 g) when compared with the total flavonoid content of 28.42 ± 0.44 mg/100g and total phenolic content of 40.38 ± 0.19 mg/100 g.
Table 4: Total antioxidant capacity of methanol extract of R. beninensis
|
Total Antioxidant Capacity (mg/100g) |
Total Flavonoid (mg/100g) |
Total Phenol (mg/100g) |
MRB |
42.11 ± 1.13 |
28.42 ± 0.44 |
40.38 ± 0.19 |
Antimicrobial activity
The antibacterial activity of the extract was presented in Table 5. The result indicated that the extract inhibited the isolates at minimum inhibitory concentrations (MICs) and minimum bactericidal concentrations (MBCs) ranging from 3.125 – 50 mg/mL and 6.25 – 25 mg/mL, respectively.
Table 5: Antimicrobial activity of methanol extract of R. beninensis
Organism code |
Antibacterial activity of R. beninensis |
|
MIC (mg/ml) |
MBC (mg/ml) |
|
E. coli EC3AT |
12.5 |
25 |
P. mirabilis EC28T |
6.25 |
12.5 |
A. indicus KB45AT |
6.25 |
12.5 |
K. pneumoniae KB24AT |
6.25 |
12.5 |
A. indicus EC41TT |
6.25 |
12.5 |
P. aeruginosa PA29T |
3.125 |
6.25 |
M. morganii PA17T |
6.25 |
12.5 |
P. aeruginosa PA129AT |
3.125 |
6.25 |
S. marcescens PA18T |
12.5 |
12.5 |
P. putida PA25T |
>50 |
ND |
KEYS: MICs = Minimum Inhibitory Concentrations, MBCs = Minimum Bactericidal Concentrations, ND = Not determined
Discussion
Using medicinal plants to treat a variety of infections, including UTIs, has received increasing attention in recent years. Consequently, seventy percent of the population of the developing world use traditional systems of healthcare39,90. Although various antibiotics have been administered to patients, it is necessary to seek for and use “non-antibiotic” approaches for therapeutic purposes due to development of antibiotic resistance, which is a somber concern in the worldwide healthcare arena91. In order to efficiently treat bacterial infections, particularly urinary tract infections, it is essential to use bioactive compounds possessing therapeutic efficacy. Numerous studies have demonstrated that plant bioactive compounds may meaningfully add to the development of new and potent medications that can modify bacterial resistance as an alternate and complementary method of addressing microbial resistance23,24,92.
In this study, the phytochemical analysis of the root extract of R. beninensis revealed that reducing sugar, alkaloids, and terpenoids were found in higher concentrations than phlobatannins, tannins, and saponins. These phytochemicals ensure diverse biological effects including antioxidant activity93. The relative conformation of phytochemicals obtained diverges from phytochemicals in R. beninensis stem extract as reported by Lasisi et al.31. The differences could result from dissimilarities in the phytochemical concentrations in different parts of the plant94,95. However, the existence of these chemical constituents in methanol root extract of R. beninensis suggests that, if judiciously screened, the studied plant could provide pharmacologically significant plant-derived pharmaceuticals. This could further bolster the fact that different parts of this plant have been employed in ethnomedicine in the treatment of a number of diseases. Alkaloids are nitrogen-based compounds and are known to have antimalarial, antihypertensive, antiarrhythmic, and anticancer effects96 and work as potent analgesics and stimulants for the central nervous system97. While saponins have antimalarial properties98, antioxidant, anti-inflammatory, anticancer, antiallergic, and antiplatelet activities have been reported for flavonoids99. The availability of these biologically important chemical compounds in R. beninensis, therefore, underscores its medicinal values. Thus, the therapeutic potential of various extracts of R. beninensis may be due to these phytochemical compounds.
The therapeutic properties of this plant species are influenced by the different bioactive compounds identified in the methanol extracts of R. beninensis. While several prominent bioactive compounds from this extract included (Z,Z)-9,12-Octadecadienoic acid (19.96%), Lupeol (18.96%), cis-13-Octadecenoic acid (9.96%), n-Hexadecanoic acid (9.87%), 3′,5′-Dimethoxyacetophenone (6.67%), and 4-((1E)-3-Hydroxy-1-propenyl)-2 -methoxyphenol (3.21%) have been known to have various inhibitory effects and therapeutic uses, the pharmacological activities of some chemical compounds identified in this study have been corroborated by Fagbemi et al.24 who identified 36 bioactive compounds and many of them possessed antioxidant, antibacterial, antiasthma, antifungal, antidiabetic, anti-cancer and anti-inflammatory activities. Kuete100 and Dzotam & Kuete101 indicated that antimicrobial activities of studied Cameroonian plants depended on the occurrence of alkaloids, flavonoids, phenolics, steroids and triterpenes. While the antimicrobial activity of methanol extracts of Artemisia vulgaris, Cinnamomum tamala, Oxalis corniculata, and Ageratina adenophora was against Klebsiella pneumoniae, Staphylococcus aureus, Escherichia coli, Citrobacter koseri and Salmonella typhi102, hydromethanol extracts of Cistus monspeliensis, Punica granatum and Berberis vulgaris indicated tremendous antibacterial activity against Enterococcus faecalis, Staphylococcus aureus, and Enterobacter cloacae103. These identified phytochemicals in the extract are believed to be a constituent of defense mechanisms in plant, and they can be categorized as protective substances present in this plant as “phytoanticipins” and “phytoprotectants”104. Despite the low percentages of some of the detected chemical compounds in this study, scientific reports indicated that each of the compounds had considerable medicinal importance, and the presence of the identified chemical compounds may have an impact on the antibacterial and antioxidant activities of R. beninensis. The chemical compounds identified underscore the veracity of this plant’s usefulness in traditional medicine while identified compounds without biological activities found in literature could also contribute individually or synergistically to the pharmacological activities of the extract.
Disproportionate free radical production damages biomolecules during cellular metabolism causing few disorders including diabetes, cancer, chronic inflammation, and neurodegenerative illnesses105. The most practical method in managing oxidative stress–related diseases is antioxidant therapy106. Despite the availability of various synthetic medications for managing oxidative stress, their high costs and unfavorable side effects have reduced their effectiveness107. As a result, alternative, low-cost, non-toxic antioxidants are required to fight oxidative stress and the diseases associated with it106. Consequently, the high potency and effectiveness at low concentrations exhibited by the methanol extract of R. beninensis implied that its redox properties are dependent on its polyphenolic contents satiating singlet and triplet oxygen and decomposing peroxides, adsorbing and neutralizing free radicals108 and directly scavenging free radicals109.
The methanol extract of R. beninensis roots was more effective against Pseudomonas spp. PA29T. At low concentrations, strong antibacterial activity was recorded against all the Gram-negative bacteria. The MBC values revealed that the extract could be more bactericidal against the Gram-negative bacteria. This was in consonance with the findings of Semwal et al. 110, Manivannan et al.111, and Kouadri112. While antibacterial agents with lower MICs and MBCs are thought to be more effective, Tripathi113 indicated that antibacterial agents are more bactericidal when the MIC is closer to the MBC. However, the variations in bacterial susceptibility could be attributed to the differences in microorganisms’ intrinsic tolerance or the physico-chemical properties of phytochemicals contained in plant extracts112 and phospholipid membranes that contain structural lipopolysaccharide components making their cell walls impermeable to antimicrobials114. While the antibacterial activity of the methanol extract could depend on the most prominent chemical compounds, the therapeutic potential of the chemical compounds with lesser percentages and those without pharmacological activities identified in literature could not be undermined.
Conclusion
In conclusion, this study confirmed the presence of different phytochemical compounds and chemical compounds with diverse pharmacologically important principles or activities in the aqueous methanol extract of R. beninensis roots. The extract’s antioxidant and antibacterial effects against the test bacterial isolates might be accredited to the occurrence of the aforementioned chemicals found in the extract. This study demonstrates the medicinal value of R. beninensis‘ root, identifies the plant as a significant source of innovative drug compounds, and suggests that this plant may be a viable alternative for treating urinary tract infections, supporting its use in the folkloric treatment of these diseases. Further studies on isolation of bioactive compounds of therapeutic values to determine their biological activities are ongoing in our research laboratory.
References
- Kalal BS, Nagaraj S. Urinary tract infections: A retrospective, descriptive study of causative organisms and antimicrobial pattern of samples received for culture, from a tertiary care setting. Germs 6(4): 132–138 (2016).
- Shaheen G, Akram M, Jabeen F, Muhammad S,Shah A, Munir N, Daniyal M, Riaz M, Mahmood I, Aymen T, Ghaur O, Sultana S, Zainab R, Khan M. Therapeutic potential of medicinal plants for the management of urinary tract infection: A systematic review. Clinical and Experimental Pharmacology and Physiology, 46(7): 613– 624 (2019).
- Al-Badr A, Al-Shaikh G. Recurrent urinary tract infections management in women: a review. Sultan Qaboos Univ. Med. J. 13(3), 359-362 (2013).
- Alteri CJ, Hagan EC, Sivick KE, Smith SN, Mobley H. Mucosal immunization with iron receptor antigens protects against urinary tract infection. PLoS Pathog. 5(9): e1000586 (2009).
- Pitart C, Marco F, Keating TA, Nichols WW, Vila J. Activity of ceftazidime-avibactam against fluoroquinolone-resistant Enterobacteriaceae and Pseudomonas aeruginosa. Antimicrob. Agents Chemother. 59(6): 3059-3065 (2015).
- Vohra P, Mengi S, Bunger R, Garg S, Pathania D, Singh VA. Bacterial uropathogens in urinary tract infection and their antibiotic susceptibility pattern in a tertiary care hospital. Int. J. Curr. Microbiol. Appl. Sci. 4(10): 696-699 (2015).
- Chiu CC, Lin TC, Wu RX, Yang YS, Hsiao PJ, Lee Y, Lin JC, Chang FY. Etiologies of community-onset urinary tract infections requiring hospitalization and antimicrobial susceptibilities of causative microorganisms. J. Microbiol. Immunol. Infect. 50(6): 879–885 (2017).
- Kajihara T, Yahara K, Stelling J, Eremin SR, Tornimbene B, Thamlikitkul V, Hirabayashi A, Anzai E, Wakai S, Matsunaga N. Comparison of de-duplication methods used by WHO Global Antimicrobial Resistance Surveillance System (GLASS) and Japan Nosocomial Infections Surveillance (JANIS) in the surveillance of antimicrobial resistance. PLoS ONE, 15(6): e0228234 (2020).
- Kang CI, Kim J, Park DW, Kim BN, Ha US, Lee SJ, Yeo JK, Min SK, Lee H, Wie SH. Clinical Practice Guidelines for the Antibiotic Treatment of Community-Acquired Urinary Tract Infections. Infect. Chemother. 50(1): 67–100 (2018).
- Werneburg GT, Henderson NS, Portnoy EB, Sarowar S, Hultgren SJ, Li H, Thanassi DG. The pilus usher controls protein interactions via domain masking and is functional as an oligomer. Nat. Struct. Mol. Biol. 22(7): 540-546 (2015).
- O’Brien VP, Hannan TJ, Nielsen HV, Hultgren SJ. Drug and vaccine development for the treatment and prevention of urinary tract infections. Microbiol. Spectr. 4: 42 (2016).
- Simpson BW, May JM, Sherman DJ, Ruiz N, Kahne D. Lipopolysaccharide transport to the cell surface: periplasmic transport and assembly into the outer membrane. Phil. Trans. Royal Soc. B: Biol. Sci. 370(1679): 20150027 (2015).
- Aguiniga LM, Yaggie RE, Schaeffer AJ, Klumpp DJ. Lipopolysaccharide domains modulate urovirulence. Infect. Immun. 84(11): 3131-3140 (2016).
- Zhang G, Meredith TC, Kahne D. On the essentiality of lipopolysaccharide to Gram-negative bacteria. Curr. Opin. Microbiol. 16(6): 779-785 (2013).
- Magyar A, Köves B, Nagy K, Dobák A, Arthanareeswaran VKA, Bálint P, Wagenlehner F, Tenke P. Spectrum and antibiotic resistance of uropathogens between 2004 and 2015 in a tertiary care hospital in Hungary. J. Med. Microbiol. 66(6): 788-797 (2017).
- Sweileh WM, Al-Jabi SW, Sa’ed HZ, Sawalha AF, Abu-Taha AS Global research output in antimicrobial resistance among uropathogens: A bibliometric analysis (2002–2016). J. Global Antimicrob. Res. 13: 104-114 (2018).
- Habte TM, Dube S, Ismail N, Hoosen AA. Hospital and community isolates of uropathogens at a tertiary hospital in South Africa. South Afr. Med. J. 99(8) (2009).
- Friedman ND, Temkin E, Carmeli Y. The negative impact of antibiotic resistance. Clin. Microbiol. Infect. 22(5): 416-22 (2016).
- El Bouamri MC, Arsalane L, El Kamouni Y, Zouhair S. Antimicrobial susceptibility of urinary Klebsiella pneumoniae and the emergence of carbapenem-resistant strains: A retrospective study from a university hospital in Morocco, North Africa. Afri. J. Urol. 21(1): 36-40 (2015).
- Erdem I, Ali RK, Ardic E, Omar SE, Mutlu R, Topkaya AE. Community-acquired lower urinary tract infections: Etiology, antimicrobial resistance, and treatment results in female patients. J. Global Infect. Dis. 10(3): 129 (2018).
- Olajuyigbe OO, Adedayo O, Coopoosamy RM. Antibacterial Activity of Defatted and Nondefatted Methanolic Extracts of Aframomum melegueta K. Schum. against Multidrug-Resistant Bacteria of Clinical Importance. The Sci. World J. (2020).
- Wintola OA, Olajuyigbe AA, Afolayan AJ, Coopoosamy RM, Olajuyigbe OO. Biological activities and chemical composition of essential oil from Erythrina caffra Thunb. used in treatment of infections and complications associated with gastrointestinal infections in South Africa. Heliyon (Elsevier) – 7(6): e07244 (2021).
- Adeoye-Isijola MO, Jonathan SG, Coopoosamy RM, Olajuyigbe OO. Molecular characterization, gas chromatography mass spectrometry analysis, phytochemical screening and insecticidal activities of ethanol extract of Lentinus squarrosulus against Aedes aegypti (Linnaeus). Mol. Biol. Rep. 48(1): 41-55 (2021).
- Fagbemi KO, Aina DA, Adeoye-Isijola MO, Naidoo KK, Coopoosamy RM, Olufunmiso Olusola Olajuyigbe OO. Bioactive compounds, antibacterial and antioxidant activities of methanolextract of Tamarindus indica Linn. – Sci. Rep. 12:9432 (2022).
- Mehta SR, Yusuf S, Peters RJ, Bertrand ME, Lewis BS, Natarajan MK, Malmberg K, Rupprecht HJ, Zhao F, Chrolavicius S. Clopidogrel in Unstable Angina to Prevent Recurrent Events Trial (CURE) Investigators. Effects of pretreatment with clopidogrel and aspirin followed by long-term therapy in patients undergoing percutaneous coronary intervention: the PCI-CURE study. The Lancet 358(9281): 527-533 (2001).
- Olajuyigbe OO, Afolayan AJ. Pharmacological assessment of the medicinal potential of Acacia mearnsii De Wild.: Antimicrobial and Toxicity activities. International J. Mol. Sci. 13(4): 4255-67 (2012).
- Almabruk KH, Dinh LK, Philmus B. Self-resistance of natural product producers: past, present, and future focusing on self-resistant protein variants. ACS Chem.Biol. 13(6): 1426-37 (2018).
- Ruddaraju LK, Pammi SV, sankar Guntuku G, Padavala VS, Kolapalli VR. A review on antibacterials to combat resistance: From ancient era of plants and metals to present and future perspectives of green nano technological combinations. Asian J. Pharma. Sci. 15(1): 42-59 (2020).
- Ojokoh AO, Mogaji O, Adeleke BS. Antimicrobial Efficacy of Chromolaena odorata Extract against Bacteria Isolated from Patients with Urinary Tract Infection in Ondo State, Nigeria. Int. J. Pathog. Res. 1(2): 1-10 (2018).
- Evuen UF, Apiamu A, Okolie NP, Orji BO. Protective activity of root extract of Rhaphiostylis beninensis against carbon tetrachloride-induced hepatotoxicity in Wistar rats. Biokemistri 27: 32(2) (2021).
- Lasisi AA, Folarin OM, Dare EO, Akinloye OA, Fisuyi MO. Phytochemical, antibacterial and cytotoxic evaluation of Raphiostylis beninensis [Hook F. ex Planch] stem bark extracts. Int. J. Pharma. Biol. Sci. 2(3) (2011).
- Burkil HM. The useful plants of net. Tropical Afr. 4th Edition Macmillan Press 1: 34-36 (1999).
- Odugbemi TA Textbook of Medicinal Plants in Nigeria. Tolu Press Lagos, p. 23-97 (2008).
- Adjanohoun EJ, et Technique ADCC, Ake Assi L, Floret JJ, Guinko S, Koumare M. Medecine traditionnelle et pharmacopee; contribution aux etudes ethnobotaniques et floristiques au Mali (1979).
- Adebayo-Tayo BC, Adegoke AA, Ajibesin KK. Rationalizing some medicinal plants used in treatment of skin diseases. Afr. J. Microbiol. Research 4(10): 958-63 (2010).
- Ofeimum JO, Onwukaeme DN. Evaluation of Phytochemical Constituent and Pharmalogical activity of roots of Raphiostylis beninensis (icacinaceae) J. Pharm. Sci. Pharm. Practs., 8: 85-90 (2006).
- Ofeimun JO, Mbionwu MI. Cytotoxic and Growth inhibitory activity of aqueous extracts of root and leaf of Rhaphiostylis beninensis Planch ex Benth and Pyrenacantha standtii Engl (Icacinaceae). J. Pharm. Biol. Res. 11(1): 8-14 (2014).
- Adeshina GO, Onaolapo JA, Ehinmidu JO, Odama LE. Phytochemical and antimicrobial studies of the ethyl acetate extract of Alchornea cordifolia leaf found in Abuja, Nigeria. J. Med. Plants Research 4(8): 649-58 (2010).
- Tan NA, Siddique BM, Muhamad II, Kok FS. The effect of solvents on the soxhlet extraction of omega 3 from perah oil. Jurnal Teknologi 67(4) (2014).
- Sofowora A. Phytochemical screening of medicinal plants and traditional medicine in Africa. Spectrum Books Limited. Nigeria, pp150–156 (1993).
- Trease GE, Evans WC. Pharmacognosy Macmillan, London (1978).
- Ejikeme C, Ezeonu CS, Eboatu AN. Determination of Physical and Phytochemical Constituents of some Tropical Timbers Indigenous to Niger Delta area of Nigeria. Eur. Sci. J. 10(18): 247-70 (2014).
- Ezeonu CS, Ejikeme CM. Qualitative and quantitative determination of phytochemical contents of indigenous Nigerian softwoods. New J. Sci. (2016).
- Obadoni BO, Ochuko PO. Phytochemical studies and comparative efficacy of the crude extracts of some haemostatic plants in Edo and Delta States of Nigeria. Global J. pure appl. Sci. 8(2): 203-8 (2002).
- Amadi BA, Agumuo EN, Ibegbulem CO. Research Methods in Biochemistry Supreme Publisher. Owerri, Nigeria. 130-3 (2004).
- Chen YX, Liu XY, Xiao Z, Huang YF, Liu B. Antioxidant activities of polysaccharides obtained from Chlorella pyrenoidosa via different ethanol concentrations. Int. J. Biol. Macromol. 91: 505-9 (2016).
- Otang WM, Grierson DS, Ndip RN. Phytochemical studies and antioxidant activity of two South African medicinal plants traditionally used for the management of opportunistic fungal infections in HIV/AIDS patients. BMC Compl. Alt. Med. 12(1): 1-7 (2012).
- Silva IK, Soysa P. Evaluation of phytochemical composition and antioxidant capacity of a decoction containing Adenanthera pavonina L. and Thespesia populnea L. Pharmacog. Mag. 7(27): 193 (2011).
- Murugan R, Parimelazhagan T. Comparative evaluation of different extraction methods for antioxidant and anti-inflammatory properties from Osbeckia parvifolia Arn. – An in vitro approach. J. King Saud Univ. Sci. 26(4): 267-75 (2014).
- Kattamis C, Lazaropoulou C, Delaporta P, Apostolakou F, Kattamis A, Papassotiriou I. Disturbances of biomarkers of iron and oxidant-antioxidant homeostasis in patients with beta-thalassemia intermedia. Ped. Endocrinol. Revs: PER. 8: 256-62 (2011).
- Carson CF, Hammer KA, Riley TV. Broth micro-dilution method for determining the susceptibility of Escherichia coli and Staphylococcus aureus to the essential oil of Melaleuca alternifolia (tea tree oil). Microbios. 82(332): 181-5 (1995).
- Ibibia ET, Olabisi KN, Oluwagbemiga OS. Gas chromatography-mass spectrometric analysis of methanolic leaf extracts of Lannea kerstingii and Nauclea diderrichii, two medicinal plants used for the treatment of gastrointestinal tract infections. Gas. 9(4) (2016).
- Melappa G, Prakash B. In vitro antimitotic, antiproliferative and GC-MS studies on the methanolic extract of endophytic fungi, penicillium species of Tabebuia argentea bur & k. Sch. Farmacia. 5: 301-9 (2017).
- Banakar P, Jayaraj M. GC-MS analysis of bioactive compounds from ethanolic leaf extract of Waltheria indica Linn. and their pharmacological activities. Int J Pharm Sci Res 9(5): 2005-10 (2018).
- Duke J. Dr. Duke’s Phytochemical and Ethnobotanical Databases. United States Department of Agriculture. Agricul. Research Serv, Accessed April, 27 (2004).
- Abdulhafiz F, Mohammed A, Kayat F, Bhaskar M, Hamzah Z, Podapati SK, Reddy LV. Xanthine oxidase inhibitory activity, chemical composition, antioxidant properties and GC-MS Analysis of Keladi Candik (Alocasia longiloba Miq). Mol. 25(11): 2658 (2020).
- Gopalakrishnan S, Vadivel E. GC-MS analysis of some bioactive constituents of Mussaenda frondosa Linn. Int. J. Pharma. Bio. Sci. 2(1): 313-20 (2011).
- Sosa AA, Bagi SH, Hameed IH. Analysis of bioactive chemical compounds of Euphorbia lathyrus using gas chromatography-mass spectrometry and fourier-transform infrared spectroscopy. J. Pharmacogn. Phytother. 8(5): 109-26 (2016).
- Mishra RC, Kumari R, Yadav S, Yadav JP. GC-MS Analysis and Molecular Docking Studies of Active Phytochemicals from Medicinal Plants against Malassezia globosa LIP1 (SMG1) Enzyme. Bio. Research Appl. Chem. 12(3): 2762-2775 (2021).
- Ashmawy NA, Salem MZ, Mervat EH, Abd El-Kareem MS, El-Shanhorey NA, Mohamed AA, Salem AZ. Antibacterial activity of the bioactive compounds identified in three woody plants against some pathogenic bacteria. Microb. Pathog. 121: 331-40 (2018).
- Hamid AA, Oguntoye SO, Negi AS, Ajao A, Owolabi NO. Chemical constituents, antibacterial, antifungal and antioxidant activities of the aerial parts of Cnidoscolus aconitifolius. Ife J. Sci. 18(2): 561-71 (2016).
- Arora S, Kumar G. Phytochemical screening of root, stem and leaves of Cenchrus biflorus Roxb. J. Pharmacogn. Phytochem. 7(1): 1445-50 (2018).
- Wintola OA, Afolayan AJ. Chemical constituents and biological activities of essential oils of Hydnora africana thumb. used to treat associated infections and diseases in South Africa. Appl. Sci. 7(5): 443 (2017).
- Beulah GG, Soris PT, Mohan VR. GC-MS determination of bioactive compounds of Dendrophthoe falcata (LF) Ettingsh: An epiphytic plant. Int. J. Health Sci. Res. 8: 261-9 (2018).
- Rahman MM, Ahmad SH, Mohamed MT, Ab Rahman MZ. Antimicrobial compounds from leaf extracts of Jatropha curcas, Psidium guajava, and Andrographis paniculata. The Sci. World J. (2014).
- Sunita A, Ganesh K, Sonam M. Screening and evaluation of bioactive components of Cenchrus ciliaris L. by GC-MS analysis. Int. Res. J. Pharm. 8(6) (2017).
- Nishanthini A, Mohan VR, Jeeva S. Phytochemical, FT-IR, and GC-MS analysis of stem and leaf of Tiliacora acuminata (lan.) Hook f & thomas (menispermaceae). Int J. Pharma. Sci. Res. 5(9): 3977 (2014).
- Vinuchakkaravarthy T, Sangeetha CK, Velmurugan D. Tris (2,4-di-tert-butylphenyl) phosphate. Acta Crystallogr Sect E Struct. Rep. Online, 66(9): o2207- o2208 (2010).
- Lalitha S, Parthipan B, Mohan VR. Determination of bioactive components of Psychotria nilgiriensis Deb & Gang (Rubiaceae) by GC-MS analysis. Int. J. Pharm. Phytochem Res 7(4): 802-9 (2015).
- Shareef HK, Muhammed HJ, Hussein HM, Hameed IH. Antibacterial effect of ginger (Zingiber officinale) roscoe and bioactive chemical analysis using gas chromatography mass spectrum. Orient. J. Chem. 32(2): 20-40 (2016).
- Walker J, Ley JP, Schwerzler J, Lieder B, Beltran L, Ziemba PM, Hatt H, Hans J, Widder S, Krammer GE, Somoza V. Nonivamide, a capsaicin analogue, exhibits anti‐inflammatory properties in peripheral blood mononuclear cells and U‐937 macrophages. Mol. Nutr. Food Res. 61(2): 1600474 (2017).
- Rangasamy K, Namasivayam E. In vitro antioxidant and free radical scavenging activity of isolongifolene. Asian J. Biol. Sci. 7(1): 13-23 (2014).
- Al-Marzoqi AH, Hameed IH, Idan SA. Analysis of bioactive chemical components of two medicinal plants (Coriandrum sativum and Melia azedarach) leaves using gas chromatography-mass spectrometry (GC-MS). Afr. J. Biotech. 14(40): 2812-30 (2015).
- Kim Y, Lee J. Anti‐inflammatory activity of capsaicin and dihydrocapsaicin through heme oxygenase‐1 induction in raw264.7 macrophages. J. Food Biochem. 38(4): 381-7 (2014).
- Oni JO, Akomaye FA, Markson AA, Egwu AC. GC-MS analysis of bioactive compounds in some wild-edible mushrooms from Calabar, Southern Nigeria. Eur. J. Biol. Biotech. 1(6) (2020).
- Aldakheel RK, Rehman S, Almessiere MA, Khan FA, Gondal MA, Mostafa A, Baykal A. Bactericidal and in vitro cytotoxicity of Moringa oleifera seed extract and its elemental analysis using laser-induced breakdown spectroscopy. Pharm. 13(8): 193 (2020).
- Chen JJ, Duh CY, Chen IS. Cytotoxic chromenes from Myriactis humilis. Planta Medica. 71(04): 370-2 (2005).
- Jones S, Eldahshan OA. Chemical Composition, Cytotoxic and Anti-arthritic Activities of Hexane Extracts of Certain Schinus Species. J. Pharm. Pharmacol. 9: 378-386 (2021).
- de Melo KM, de Oliveira FT, Silva RA, Quinderé AL, Marinho Filho JD, Araújo AJ, Pereira ED, Carvalho AA, Chaves MH, Rao VS, Santos FA. α, β-Amyrin, a pentacyclic triterpenoid from Protium heptaphyllum suppresses adipocyte differentiation accompanied by down regulation of PPARγ and C/EBPα in 3T3-L1 cells. Biomed Pharmacother. 109: 1860-6 (2019).
- Ching J, Chua TK, Chin LC, Lau AJ, Pang YK, Jaya JM, Tan CH, Koh HL. Beta-amyrin from Ardisia elliptica Thunb. is more potent than aspirin in inhibiting collagen-induced platelet aggregation. Indian J. Exp. Biol.48(3): 275-9 (2010).
- Otuki MF, Ferreira J, Lima FV, Meyre-Silva C, Malheiros A, Muller LA, Cani GS, Santos AR, Yunes RA, Calixto JB. Antinociceptive properties of mixture of α-amyrin and β-amyrin triterpenes: evidence for participation of protein kinase C and protein kinase A pathways. J. Pharmacol. Exp. Therap. 313(1): 310-8 (2005).
- Melo CM, Morais TC, Tomé AR, Brito GA, Chaves MH, Rao VS, Santos FA. Anti-inflammatory effect of α, β-amyrin, a triterpene from Protium heptaphyllum, on cerulein-induced acute pancreatitis in mice. Inflamm. Research. 60(7): 673-81 (2011).
- Ching J, Lin HS, Tan CH, Koh HL. Quantification of α‐and β‐amyrin in rat plasma by gas chromatography–mass spectrometry: application to preclinical pharmacokinetic study. J. Mass Spectr. 46(5): 457-64 (2011).
- Jalill RD. Antagonistic effect between Citrullus colocynthis extract and TiO2 nanoparticles in anticancer combination therapy. Iraqi J. Cancer Med. Gen. 9(2) (2016).
- Mujeeb F, Bajpai P, Pathak N. Phytochemical evaluation, antimicrobial activity, and determination of bioactive components from leaves of Aegle marmelos. BioMed Res. Int. 1-11 (2014).
- Singariya P, Mourya KK, Kumar P. Gas Chromatography-Mass Spectrometric Analyses of acetone extract of Marwar dhamangrass for bio-active compounds. Plant Arch. 15(2): 1065-74 (2015).
- Luo X, Su P, Zhang W. Advances in microalgae-derived phytosterols for functional food and pharmaceutical applications. Marine drugs. 13(7): 4231-54 (2015).
- Reddy RA, Krishna V, Usha S, Bharathi R, Murthy VK. GC-MS analysis of methanolic extract of stem and root bark of Kirganelia reticulata for bioactive components. J. Fundam. Appl. Sci. 6: 8-13 (2017).
- Mohammed GJ, Omran AM, Hussein HM. Antibacterial and phytochemical analysis of Piper nigrum using gas chromatography-mass Spectrum and Fourier-transform infrared spectroscopy. Int. J. Pharmacogn. Phytochem. Research8(6): 977-96 (2016).
- Bodeker G. Traditional Health Knowledge and Public-Policy. Nat. Res. 30(2): 5-16 (1994).
- Daswani PG. Non-antibiotic potential of medicinal plants to combat urinary tract infections. Curr. Sci. 117(9): 1459 (2019).
- Olajuyigbe OO, Onibudo TE, Coopoosamy RM, Ashafa AO, Afolayan AJ. Bioactive compounds and in vitro antimicrobial activities of ethanol stem bark extract of Trilepisium madagascariense DC. Int. J. Pharmacol. 14(7):901-12 (2018).
- Rubió L, Motilva MJ, Romero MP. Recent advances in biologically active compounds in herbs and spices: a review of the most effective antioxidant and anti-inflammatory active principles. Crit. Rev. Food Sci. Nutrit.53(9): 943-53 (2013).
- Kouki M, Manetas Y. Resource availability affects differentially the levels of gallotannins and condensed tannins in Ceratonia siliqua. Biochem. Syst. Ecol. 30(7): 631-9 (2002).
- Monteiro JM, Albuquerque UP, Lins Neto EM, Araújo EL, Albuquerque MM, Amorim EL. The effects of seasonal climate changes in the Caatinga on tannin levels in Myracrodruon urundeuva (Engl.) Fr. All. and Anadenanthera colubrina (Vell.) Brenan. Revista Brasileira de Farmacognosia.16: 338-44 (2006).
- Heikens J, Fliers E, Endert E, Ackermans M, Van Montfrans G. Liquorice-induced hypertension—a new understanding of an old disease: case report and brief review. The Netherlands J. Med.47(5): 230-4 (1995).
- PAshok PK, Upadhyaya K. Tannins are astringent. J. Pharmacogn. Phytochem. 1(3): 45-50 (2012).
- Besong EE, Balogun ME, Djobissie SF, Mbamalu OS, Obimma JN. A review of Piper guineense (African black pepper). Int. J. Pharm. Pharm. Res, 6: 368-84 (2016).
- Pal DI, Verma PR. Flavonoids: A powerful and abundant source of antioxidants. Int. J. Pharm. Pharmaceut. Sci. 5(3): 95-8 (2013).
- Kuete V. Potential of Cameroonian plants and derived products against microbial infections: a review. Planta Medica. 76(14): 1479-91 (2010).
- Dzotam JK, Kuete V. Antibacterial and antibiotic-modifying activity of methanol extracts from six Cameroonian food plants against multidrug-resistant enteric bacteria. BioMed Res. Int. (2017).
- Manandhar S, Luitel S, Dahal RK. In vitro antimicrobial activity of some medicinal plants against human pathogenic bacteria. J. Trop. Med. 1-5 (2019).
- Bereksi MS, Hassaïne H, Bekhechi C, Abdelouahid DE. Evaluation of antibacterial activity of some medicinal plants extracts commonly used in Algerian traditional medicine against some pathogenic bacteria. Pharmacogn J. 10(3) (2018).
- Salehi B, Ata A, V Anil Kumar N, Sharopov F, Ramírez-Alarcón K, Ruiz-Ortega A, Abdulmajid Ayatollahi S, Tsouh Fokou PV, Kobarfard F, Amiruddin Zakaria Z, Iriti M, Taheri Y, Martorell M, Sureda A, Setzer WN, Durazzo A, Lucarini M, Santini A, Capasso R, Ostrander EA; Atta-ur-Rahman, Choudhary MI, Cho WC, Sharifi-Rad J. Antidiabetic Potential of Medicinal Plants and Their Active Components. Biomolecules. 9(10): 551 (2019).
- Zhou L, Chen P, Peng Y, Ouyang R. Role of oxidative stress in the neurocognitive dysfunction of obstructive sleep apnea syndrome. Oxid. Med. Cell. Longev. Article ID 9626831 (2016).
- Liu Z, Ren Z, Zhang J, Chuang CC, Kandaswamy E, Zhou T, Zuo L. Role of ROS and nutritional antioxidants in human diseases. Front. Physiol. 9: 477 (2018).
- Lourenço SC, Moldão-Martins M, Alves VD. Antioxidants of natural plant origins: From sources to food industry applications. Molecules (22): 4132 (2019).
- Zheng W, Wang SY. Antioxidant activity and phenolic compounds in selected herbs. J. Agricultur. Food chem.49(11): 5165-70 (2001).
- Sandhar HK, Kumar B, Prasher S, Tiwari P, Salhan M, Sharma P. A review of phytochemistry and pharmacology of flavonoids. Int. Pharmaceut. Sci. 1(1): 25-41 (2011).
- Semwal RB, Semwal DK, Combrinck S, Cartwright-Jones C, Viljoen A. Lawsonia inermis L.(henna): Ethnobotanical, phytochemical and pharmacological aspects. J. Ethnopharmacol. 155(1): 80-103 (2014).
- Manivannan R, Aeganathan R, Prabakaran K. Anti-microbial and anti-inflammatory flavonoid constituents from the leaves of Lawsonia inermis. J. Phytopharmacol. 4: 212-6 (2015).
- Kouadri F. In vitro antibacterial and antifungal activities of the saudi Lawsonia inermis extracts against some nosocomial infection pathogens. J. Pure Appl. Microbiol. 12(1): 281-6 (2018).
- Tripathi AM, Tiwary BN. Biochemical constituents of a wild strain of Schizophyllum commune isolated from Achanakmar-Amarkantak Biosphere Reserve (ABR), India. World J. Microbiol. Biotechnol. 29(8): 1431–1442 (2013).
- Leach LC. Structural elucidation of some antimicrobial constituents from the leaf latex of aloe. BMC Compl. Altern. Med. 15(270): 1-7 (2015).